ABOVE: © ISTOCK.COM, THOMASVOGEL
When two distinct liquids emerge from what was once a mixed solution—such as when oil and vinegar separate in a salad dressing—physicists call the process liquid-liquid phase separation (LLPS). Condiments aside, this phase separation also happens on the microscopic scale, inside cells, where it concentrates biomolecules and facilitates their reactions. While there are several examples of LLPS in eukaryotic cells, the process has been less extensively studied in bacteria because of their tiny size. Consequently, questions remain as to when, how and why LLPS occurs in prokaryotes.
Thanks to a report in Science Advances yesterday (October 20), “LLPS is now . . . firmly confirmed in prokaryotic systems,” Frederic Rousseau, who studies protein aggregation at KU Leuven in Belgium and was not involved in the research, writes in an email to The Scientist. It underlines “how fundamental LLPS is to cellular life and maybe...
See “These Organelles Have No Membranes”
Traditional microscopy methods have revealed protein aggregations suspected to arise by LLPS—so-called condensates—in bacteria, says chemist and biophysicist Julie Biteen of the University of Michigan who also was not a part of the research team, but understanding the mechanics of their formation and whether they are liquid or solid “has been a real challenge,” she says. This is in part because the condensates are only marginally larger, if at all, than the microscopy diffraction limit—the minimal distance at which two objects can be seen as separate, or resolved (around 200 nm for fluorescence microscopes). The new paper is thus “a really important . . . demonstration of how state-of-the-art super-resolution imaging can be used for these problems.”
Biophysicists Fan Bai of Peking University and Mark Leake of the University of York had previously shown that protein condensates, which they call aggresomes, form within Escherichia coli cells when the bacteria are stressed by antibiotic treatment or a reduction in the availability of ATP, a form of molecular energy. In the team’s latest study, they investigated the dynamics of aggresome formation as well as their function.
Using fluorescently labeled proteins that the team had previously shown accumulate in aggresomes, the researchers found that upon ATP depletion, the aggresomes form gradually over several hours, normally resulting in one at each end of the rod-shaped bacteria. With a special type of fluorescence microscopy called rapid super-resolved single-molecule tracking, which follows the movements of individual labeled proteins, they also showed that within the aggresomes the proteins freely diffuse in a way that suggests they’re moving through a liquid environment.
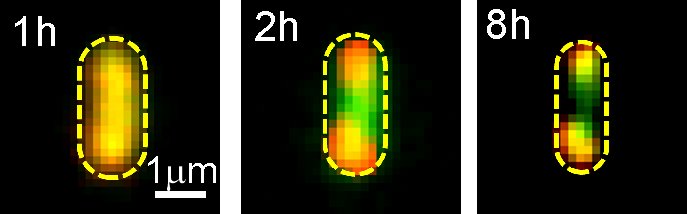
Further evidence that the aggresomes are liquid came from experiments showing they could be dissolved by an organic solvent that cannot dissolve solids and that fluorescently labeled proteins diffuse from one half of an aggresome to the other. Additionally, computer simulations showed, among other things, that rather than being an active process, no input of energy was required for aggresome formation—that is, the globs of proteins develop “at thermal equilibrium,” says Leake, which is indicative of true LLPS.
Although the team does not yet know how the aggresomes form, they suspect it might have something to do with ATP itself. The molecule is a hydrotrope, Leake explains, meaning it has the ability to make hydrophobic molecules, including some proteins, soluble in aqueous environments. Thus, its depletion might spur hydrophobic proteins to gather together.
Regardless of the mechanism, “the major finding of our paper is to show that in aggresomes proteins tend to stay together but not rigidly together. They still show a certain degree of movement. That is why we call it a liquid protein droplet, not a solid protein ball,” writes Bai in an email to The Scientist.
So, why does it matter if an aggresome is solid or liquid?
“It tells you about how they form and what sort of molecular interactions drive them to assemble,” says Stephanie Weber of McGill University who studies the spatial organization of cells and was not involved in the research. If they were solid, they would be less dynamic, limiting the types of interactions or reactions the proteins within them could perform, she explains. Also, compared with solid structures, liquid droplets can form and dissolve quickly, explains Leake, so for bacteria that live fast, replicate fast, and may experience sudden changes to their surroundings such as antibiotics or other forms of stress, “this might be a clever strategy to align with the timescale of their rapidly changing environment.”
The team went on to show that these aggresomes were not unique to E. coli, being formed in response to reduced ATP in eight other species of gram negative bacteria, and that the aggresomes promoted cell survival. Mutant E. coli that were unable to form aggresomes (found via screening a library of mutant strains), but whose viability was otherwise seemingly unaffected, had poorer survival when ATP was depleted compared to controls. Moreover, because the mutations only impaired aggresome formation and did not completely eradicate it, the team could see that “if [a mutant cell] had observable aggresomes, they were more likely to survive” than those that didn’t, says Leake.
“Showing not only that condensates form in bacteria, but that they have a functional effect on survival and stress resistance, I think is really exciting,” Weber says. However, as well as figuring out the mechanism that triggers the LLPS, knowing exactly what proteins are contained within the liquid globs would be key, she says. “That is the million-dollar question.”
Interested in reading more?
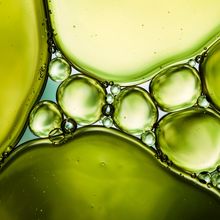