© WORAKIT/ISTOCKPHOTO.COM
Sarah “Sally” Otto was sitting in a lab meeting of evolutionary biologist Marcus Feldman’s group at Stanford University in 1988 when she overheard a graduate student describe sex as “such a big puzzle.” Otto, an undergraduate at the time, didn’t agree. Sexual reproduction—genetic recombination between individuals—“obviously” promotes variation, she thought, allowing species to adapt to changing environments.
WHY SEX?: See full infographic: JPG | PDFOtto’s reaction makes sense, and echoes one of the oldest formal explanations for why sex evolved to be ubiquitous in the animal kingdom. In 1886, German evolutionary biologist August Weismann proposed that sexual reproduction reshuffles genes to create “individual differences” upon which natural selection acts. Additional ideas have emerged since Weismann’s hypothesis: sex rids the genome of deleterious mutations; sex rapidly introduces beneficial mutations; sex helps organisms dodge parasitic infections. Yet these evolutionary justifications for sex have remained hypotheses because there is...
“Sexual reproduction permeates biology at every level, and yet it’s not intuitively obvious why it should be that way, because the costs are so high,” says Aneil Agrawal, an evolutionary geneticist at the University of Toronto.
More than 99 percent of multicellular eukaryotes reproduce sexually and have evolved elaborate ways to do so, including behavioral, physiological, and biochemical adaptations. So there must be some enduring benefit. But despite years of observing, theorizing, and experimenting, researchers have been unable to pin down exactly what that might be. “Why sex evolved is very hard to answer,” says Timothy James, who studies sex in fungi at the University of Michigan in Ann Arbor. “[Many] evolutionary biologists are trying to understand why it’s so rarely lost, even though it’s so costly.”
One reason for the continued mystery may be scientists’ choice of study organisms—typically familiar animals with unremarkable sex lives. “We know a lot about sex in humans and mice and Drosophila,” says Otto, now a theoretical biologist at the University of British Columbia who studies how sex evolved. But to better understand the pros and cons of sexual reproduction, the best place to look may be the outliers, such as eukaryotic organisms that have weird sex, or those that have no sex at all.
Today, research on such outliers is happening in force. A new batch of species is pushing researchers closer to resolving the paradox of sex, providing some of the first experimental evidence for and against ideas such as Weismann’s hypothesis. Recent studies using freshwater rotifers and genetically engineered yeast, for example, suggest sex is critical in times when environmental stress requires adaptation, while parasite-battling snails make the case that sex evolved as a powerful defense against infection.
“We are at a stage where we are pushing the boundaries, both in computer simulations and in experiments,” says Otto. “It’s not hopeless. There is movement in the field.”
Finding a partner
Most model organisms, such as mice and flies, are not useful for experiments related to the evolution of sex because they don’t reproduce both sexually and asexually. “If you want to test the effect of sex, you need to hold everything constant apart from the presence or absence of sex,” says Matthew Goddard of the University of Auckland in New Zealand. “Most higher organisms have to have sex to reproduce, so they’re out. Bacteria only reproduce asexually, so they’re out.” But there are a handful of species that fit the bill.
CHANGING WITH THE TIMES: In the lab, yeast that reproduce sexually adapt more rapidly to harsh environmental conditions and survive better than asexual strains, supporting Weismann’s hypothesis. Asexual yeast also appear to be stunted by accumulating deleterious mutations, suggesting that Muller’s ratchet also impacts yeast evolution.
See full infographic: JPG | PDF © KIMBERLY BATTISTAYeast reproduce asexually—via either budding or fission—in the presence of plentiful food. When food is scarce, however, they undergo meiosis and form haploid spores that eventually fuse with other spores to form a diploid zygote. (See illustration.) But the starvation process is mutagenic in yeast, making it impossible to isolate the effects of sex. In 2005, when Goddard was a postdoc at Imperial College London, he and his colleagues found a way to sidestep the problem: genetically engineer yeast that continue to reproduce asexually when starved. This allowed the researchers to compare starved populations of asexual yeast with starved populations of a sexually reproducing wild-type strain.
The team plopped both strains into benign and harsh conditions, measured the fitness of the populations, and got a straightforward result: sex offered no benefit in the benign environment—there was no difference in fitness between the two populations—but in the harsh environment, sexual populations adapted more rapidly and survived better than the asexuals.1 “Lo and behold, our data were in line with Weismann’s central idea,” says Goddard. “It showed that sex increased the rate of adaptation compared to asexual populations.”
Goddard’s team then went a step further to test two other theoretical predictions about sex, made by American geneticist and Nobel laureate Hermann Muller and English statistician and geneticist Ronald Fisher. The Fisher-Muller hypothesis, developed by the two thinkers independently, proposes that sex is a means of rapidly introducing beneficial mutations into a population, while Muller’s “ratchet” hypothesis suggests that sex is necessary for purging the genome of deleterious mutations. By deleting a gene involved in DNA repair, Goddard created strains of both asexual and sexual yeast with increased mutation rates, then compared those strains to normally mutating yeast under benign and stressful conditions.
This time, the data weren’t so clear-cut. But in general, “we came to the conclusion that it was both,” says Goddard: the sexual species appeared to gain beneficial mutations and adapt better than asexuals, supporting the Fisher-Muller hypothesis, and the fitness of asexuals seemed stunted by increased mutation loads, succumbing to Muller’s ratchet.2
BECOMING MORE SEXUAL: Researchers have found that a higher rate of sexual reproduction is favored in Brachionus calyciflorus rotifers when the animals are in spatially heterogeneous environments or adapting to new conditions. While sex generates genotypes that are less successful than their asexual counterparts in the short term, sexual populations fare better during periods of environmental change.© KIMBERLY BATTISTAA second organism that has proven to be a fruitful laboratory species for testing theories about sex is a freshwater species of rotifer called Brachionus calyciflorus. The transparent, microscopic animal can be found in ponds and other freshwater communities, and, importantly for the study of sex, it reproduces asexually at low densities but sexually in crowded environments. (See illustration.) When bunched together, the rotifers release a chemical cue that stimulates some females to produce haploid eggs that either develop into males or are fertilized to become diploid females. The propensity for the animals to switch from asexual to sexual reproduction is genetically controlled, allowing populations to evolve to be more sexual, and allowing the University of Toronto’s Agrawal to test some key evolutionary ideas.
He and his postdoc Lutz Becks first wanted to test the spatial heterogeneity hypothesis, which suggests sex is favored in a heterogeneous environment. Within a diverse environment, gene combinations may be beneficial in one area but detrimental in another. If individuals migrate between different regions, sex could be valuable because it breaks apart maladaptive gene combinations while creating new, potentially beneficial ones. Becks designed an experiment to see if a heterogeneous environment would promote sex in rotifers. “Frankly, I didn’t think it would work,” says Agrawal. “But it turned out really well.” In homogeneous environments, with all good- or all bad-quality food, rotifer clones became overwhelmingly asexual, while an environment with mixed food sources resulted in populations that were more likely to make the switch to sex.3
Next, they tested Weismann’s hypothesis that sex promotes adaptation, by looking to see if sex is favored as rotifers adapt to new environments. After switching rotifers from one environment to another, differing in food type and sodium chloride concentration, Agrawal and Becks counted the number of offspring and assessed the genotype diversity of both sexually derived and asexually derived populations. They found that sex does generate genetic variation, but in the short term, the genotypes created by sex were on average less successful than their asexual counterparts. Some genotypes, however, were much more successful, with the result that in the long term, the rotifer lineages that were more likely to make the switch to sex fared better, blossoming during periods of environmental change.4
But Agrawal’s and Goddard’s experiments, both researchers admit, have a major limitation: they were done in a lab using a limited set of environments. “At the end of the day, we’d like to know why sex is maintained in nature,” says Agrawal. “That’s a much harder nut to crack.”
Chasing the queen
RUNNING AWAY FROM PARASITES: The evolutionary dynamics of New Zealand snails (Potamopyrgus antipodarum) lend the strongest support for the idea that escaping parasitic infection could drive the ubiquity of sexual reproduction—the Red Queen hypothesis. Threatened with infection by more than a dozen species of trematode worms, sexually reproducing P. antipodarum snails are the most successful in generating resistant offspring. Asexual clones may start to rise in frequency, but the parasites quickly evolve to infect these increasingly common genotypes, thereby driving them down in frequency once again. © KIMBERLY BATTISTAAs a postdoc at the University of Canterbury in New Zealand in the 1980s, ecologist Curt Lively picked up Graham Bell’s The Masterpiece of Nature: The Evolution and Genetics of Sexuality. In it, Lively read about a type of unusual mud snail (Potamopyrgus antipodarum) that exhibited both sexual and asexual reproduction and was studied by Mike Winterbourn, a Canterbury freshwater ecologist who happened to work just down the hall. Lively thought he’d stumbled upon the perfect system to study how organisms switch from asexual to sexual reproduction, and he hurried over to Winterbourn’s office to see about getting started. But Winterbourn explained to him that the New Zealand snails are either one or the other—they don’t change during their lifetimes. So the snails weren’t a good system to study sexual switching, but they were ideal for testing hypotheses about the maintenance of sex.
Observing the snails in New Zealand lakes and in the lab, Lively looked for evidence that sex prepares offspring for complex and competitive environments (the tangled bank hypothesis); that sex yields a range of offspring, which can better adapt to environments that change over time than asexual clones (the lottery model); and that sex is favored for unknown reasons, but abandoned when mates are hard to find (the reproductive assurance hypothesis). “I found no support for these ideas,” Lively says.
Then he looked for evidence to evaluate the Red Queen hypothesis, which posits that interactions with parasites can drive selection for sexual reproduction. The assumption is that parasites evolve to infect the most common host genotypes, and that sexual reproduction has the advantage of being more likely to produce rare resistant genotypes.
Initially, Lively thought the Red Queen hypothesis wasn’t viable because he didn’t think parasites were virulent enough to outweigh the costs of sex for the host. Also, he adds, he had no experience studying parasites, and he didn’t want to start. “It’s embarrassing,” he says. “I had a bias against that hypothesis.” Today it’s the only hypothesis left standing for the tiny New Zealand snails.
P. antipodarum snail populations are infected in nature by more than a dozen species of trematode worms. (See illustration.) Upon infection and reproduction in their hosts, the worms sterilize the snails, putting the snails under strong selection pressure to evade the worms. When Lively and his team began sampling wild snail populations in one lake in 1994, there were several asexual clones with common genotypes, but they weren’t infected. This ran contrary to the Red Queen, which predicts that parasites would go after the most common genotypes, whether they are produced sexually or asexually. But as his team continued to genotype the snails, the pattern changed: those common asexual clones became infected. “Clones common in 1994 were resistant to infection, which is probably why they were so common, but by 2001 they were highly susceptible, and had been driven down in frequency,” says Lively. “That’s very fast”—just seven generations for the annually reproducing mollusks. The researchers replicated the experiment in the lab and saw the same results: the trematode parasites evolved to infect the most common genotypes, whose prevalence subsequently dropped.
We are at a stage where we are pushing the boundaries, both in computer simulations and in experiments. It’s not hopeless. There is movement in the field.—Sarah “Sally” Otto,
University of British Columbia
Now at Indiana University in Bloomington, Lively has continued to test the Red Queen hypothesis—looking to see, for example, if clonal genotypes common in the recent past are more susceptible to infection by local populations of parasites. In work that is currently in press, his team sampled four sites in a lake over five years and determined that asexual individuals averaged across all four sites were more infected than sexual snails, usually by a large amount, in four of those years. Only in the fifth year were sexuals more infected, which Lively attributes to the parasites reducing the prevalence of the asexual clones. This April, his team showed that exposure to parasites increases both the rate of mating and the number of different mating partners for both males and females in the sexual snail populations.5
“So, over time, I’ve begun to think the Red Queen theory is probably a real driver” of the evolution of sex, says Lively. Parasites target common genotypes, encouraging host genetic recombination. Without sex, P. antipodarum snails, at least, would almost surely be extinct.
Perks of abstinence
With so many hypotheses extolling the benefits of sex, one might think asexual creatures are doomed to extinction—unless, that is, there are other ways to achieve those same benefits.
Bdelloid rotifers used to be just a quick footnote in textbooks as “animals that lost the ability to have sex.” Today, they’re a whole chapter unto themselves. Though other animal species, especially insects, occasionally experiment with total asexuality, these attempts are rarely successful. Bdelloids, on the other hand, have been successfully reproducing for more than 80 million years and have diversified into 450 different species, despite molecular evidence that they lost the ability to have sex tens of millions of years ago.
“These organisms have apparently avoided the extinction that is the inevitable consequence of giving up sex,” says David Mark Welch, an evolutionary biologist at the Marine Biological Laboratory in Woods Hole, Massachusetts. “So what are they doing that all the other asexuals tried and failed? If we can identify that, then that will give us a strong hint as to why everything else needs to have sex.”
BLOWING IN THE WIND: Bdelloid rotifers are the most successful animals that are completely asexual. But that doesn’t mean they can’t outwit their pathogenic enemies and introduce genetic novelty into the population. By ridding themselves of all their water, for example, desiccated rotifers can escape parasites simply by being blown away in the wind. Moreover, researchers believe the extreme desiccation compromises the rotifers’ cellular integrity, permitting DNA from nearby organisms to integrate into the genome. © KIMBERLY BATTISTAResearchers have failed to find evidence of Muller’s ratchet in bdelloids; the animals have no observable mechanism for avoiding the accumulation of deleterious mutations. But there is evidence supporting the Red Queen hypothesis in the unique way that the rotifers evade parasitic infections. Ridding themselves of all their water, the rotifers become as light as flecks of sand and blow away in the wind, leaving their parasites behind. (See illustration.) Christopher Wilson and colleagues at Cornell University in New York isolated three bdelloid species from moss, grew them in petri dishes with rainwater, and exposed them to a fungal parasite. When the rotifers were exposed to air, they desiccated within 24 hours, and then a light breeze from several fans blew the dried-up organisms around a wind chamber and onto fresh Petri dishes, where new populations grew—all of them infection free. The bdelloids rid themselves of six different parasites this way.6
Last year, researchers found evidence that desiccation also provides a mechanism by which the bdelloids introduce genetic variation. Desiccation is thought to compromise cellular integrity, which allows the absorption of DNA from other sources—bacteria, fungi, and other nearby organisms. “In bdelloids, something like 7 to 10 percent of the genes are of nonmetazoan origin,” says Mark Welch. That novel DNA is then put to use, it seems. Two years ago, a team of UK researchers found that most of the rotifers’ foreign genes are expressed, and some of their proteins are even used in critical processes such as breaking down toxins and resisting antibiotics.7 And if the bdelloids are picking up DNA from other organisms, they are likely also picking up DNA from each other. “That transfer may be a surrogate for the exchange that happens during meiosis,” says Mark Welch. Thus, while they may not have sex, bdelloid rotifers are diversifying nonetheless, a process that appears critical to survival on this planet.
At the end of the day, we’d like to know why sex is maintained in nature. That’s a much harder nut to crack.—Jeffrey Albaugh,
NorthShore University HealthSystem
In a static world, sex is likely unnecessary. The ever-changing environments of Earth call for a different scenario, however. Sex allows species to adapt to the loss of food sources, the arrival of parasites, rising temperatures, and more.
There is some doubt, however, whether the environment fluctuates fast enough to warrant the prevalence and persistence of sex in the eukaryotic kingdom. “Is the force favoring sex large enough in the face of the costs?” asks Otto. “The niggling doubt in the back of my head is that it is not.”
And so the paradox of sex lives on. “We still really don’t know the answer to this very most basic question,” says Mark Welch. “We don’t know why sex exists.”
Table for One Like Saccharomyces, Cryptococcus have two separate haploid mating types, a and α, but unlike the even distributions observed in other fungi, Cryptococcus populations are 99.9 percent α and 0.1 percent a, a ratio that seemingly precludes normal sex because only one of the two partners is readily available. Because of this, most researchers had believed Cryptococcus to be primarily asexual. Then Heitman observed the fungi doing something pretty strange. Clones of the same mating type, with exactly the same genome, were having sex, both in the lab1 and in the field.2 Haploid α clones fuse with other α haploids to form diploid offspring—a cell with two copies of the exact same haploid genome. It is an extreme form of inbreeding—mating with one’s identical twin—that Heitman christened “unisexual reproduction.” But why would an individual invest the time and energy to undergo meiosis, find a partner, then recombine identical genomes, instead of simply budding off an exact copy of itself through asexual reproduction? In the last year, Heitman’s team has shown that this unique sexual process has two benefits. First, it supports the switch from a yeast stage—a rounded cellular structure, good for growing in liquid—to a filamentous fungal stage, in which a pin-like structure projects upwards to release spores. This allows Cryptococcus to disperse throughout an environment, gaining access to nutrients and mating partners.3 What’s more, unisexual reproduction also suggests that the origins of sex may not have required the existence of two sexual forms, like a male and a female or an a and an α. “Given how sex works in most animals and plants, we’re fixated on the idea that sex must have involved two sexes and must have evolved to mediate genetic exchange,” says Heitman. “I’m not convinced.”
|
References
- M.R. Goddard et al., “Sex increases the efficacy of natural selection in experimental yeast populations,” Nature, 434:636-40, 2005.
- J.C. Gray, M.R. Goddard, “Sex enhances adaptation by unlinking beneficial from detrimental mutations in experimental yeast populations,” BMC Evol Biol, 12:43, 2012.
- L. Becks, A.F. Agrawal, “Higher rates of sex evolve in spatially heterogeneous environments,” Nature, 468:89-92, 2010.
- L. Becks, A.F. Agrawal, “The evolution of sex is favoured during adaptation to new environments,” PLOS Biol, 10:e1001317, 2012.
- D.M. Soper et al., “Exposure to parasites increases promiscuity in a freshwater snail,” Biol Lett, 10:20131091, 2014.
- C.G. Wilson, “Desiccation-tolerance in bdelloid rotifers facilitates spatiotemporal escape from multiple species of parasitic fungi,” Biol J Linn Soc, 104:564-74, 2011.
- C. Boschetti et al., “Biochemical diversification through foreign gene expression in bdelloid rotifers,” PLOS Genet, 8:e1003035, 2012.
Interested in reading more?
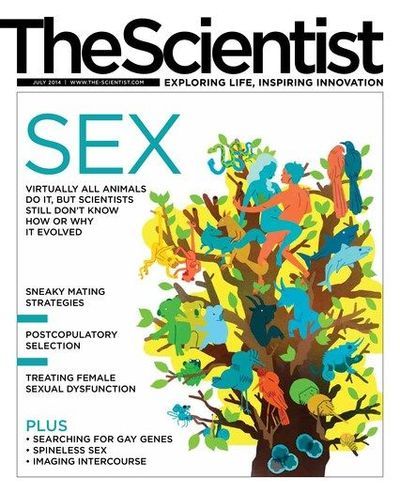