ABOVE: A new study revealed that fibrotic scarring caused by various treatments contributes directly to tumor recurrence of glioblastoma, a common and aggressive form of brain cancer. iStock, Nur Ceren Demir
In 2016, cancer biologist Johanna Joyce of the Memorial Sloan Kettering Cancer Center published a study in Science that examined the effects of a macrophage-targeted therapy on glioblastoma multiforme (GBM), a common and aggressive brain tumor.1 She found that in preclinical mouse models, GBM tumors regressed in response to the therapy, but with mixed long-term results. About half the time, the tumors would recur. In the other half, they remained in a fully dormant state.
“In every case where we found a recurrence of the tumor, this was always adjacent to a scar that had formed in the brain.” Joyce said. “This really made us think, is this causal or consequential?”
In a recent study published in Cancer Cell, Joyce, now at the University of Lausanne, revealed the findings from her team’s investigation into this question.2 The researchers discovered that fibrotic scar tissue formed after macrophage-targeted treatment acts as a safe harbor for dormant cancer cells and actively contributes to recurrence of resistant GBM. More importantly, they found that the process of scar formation—and therefore, tumor recurrence—can be stopped.
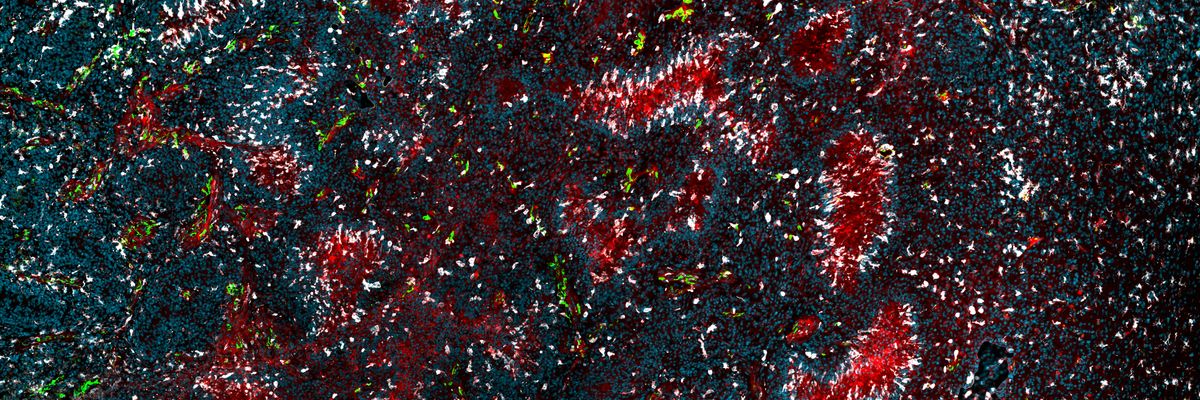
“This research is crucial because glioblastoma remains one of the most difficult cancers to treat, with a high rate of recurrence despite intensive therapies. The study sheds light on the fibrosis-driven mechanisms that enable tumor cells to survive post treatment,” Misty Jenkins, an immunologist at the Walter and Eliza Hall Institute of Medical Research who studies brain cancer and was not involved in the research, wrote in an email.
To map out the cellular and molecular terrain of the scar tissue, Joyce and her team applied an integrated multiomics approach to the scar tissue: mass spectrometry-based proteomics, single-cell RNA sequencing, and spatial transcriptomics.
Given the fibrotic nature of the scars, they reasoned that extracellular matrix (ECM) proteins would be prominent. “We indeed found that many different extracellular matrix proteins—many collagens, fibronectin…and so forth—were enriched in the scar,” Joyce remarked.
Joyce and her team developed a machine learning model that allowed them to integrate the results of sequential immunofluorescence experiments conducted across a range of time points post treatment with the omics data. “By doing this in a time course, in a dynamic manner, that really allowed us to identify the key networks within the tumor microenvironment, the cell types that appeared to be the nodes of those networks, how that changed in response to the therapeutic intervention, and how in the context of recurrence, those networks were completely restructured,” Joyce elaborated.
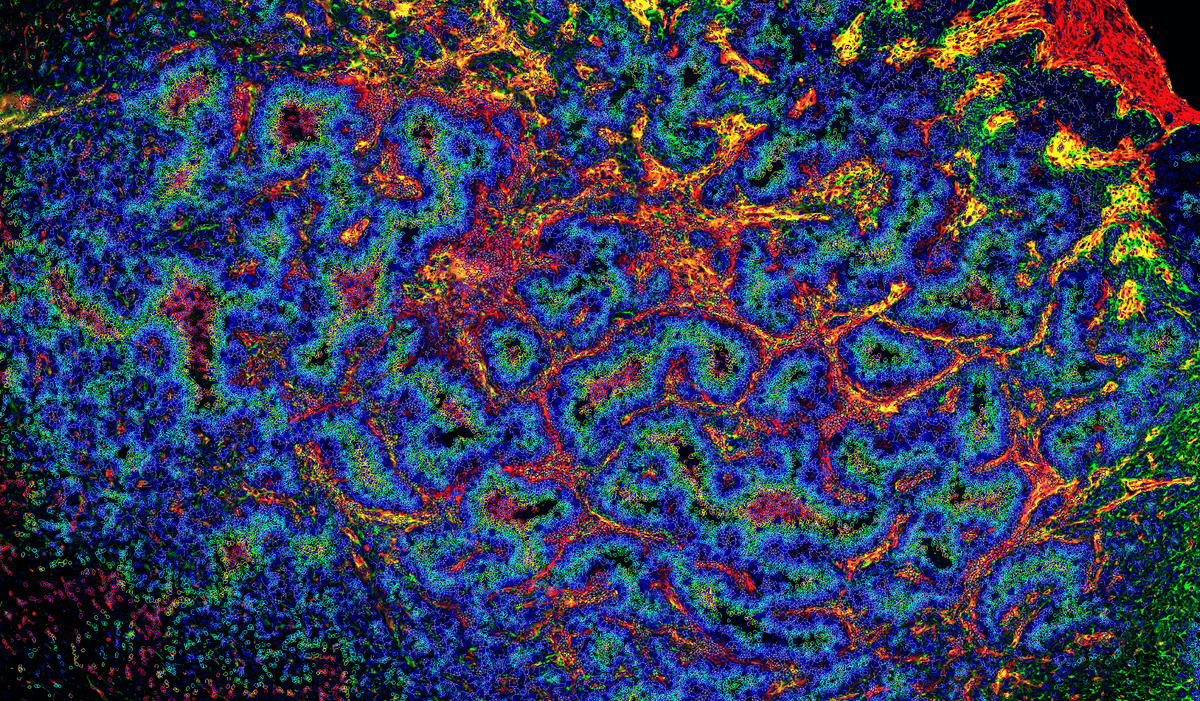
The results revealed that fibroblast-like cells predominantly drove fibrosis and scar formation in post-treatment tissue, gradually fanning out over the region where the tumor used to be and secreting the ECM proteins. The resulting scar acts as a protumor survival niche, harboring any remaining cancer cells and shielding them from the immune system, but keeping them temporarily in a non-proliferative state.
By labeling the tumor cells in genetically engineered mice with green fluorescent protein (GFP), Joyce and her colleagues observed how tumors regress and recur. In some cases, even a single GFP-positive tumor cell could be seen lying in wait in the scar tissue for many months before emerging, reactivating proliferation programs and causing tumors to rear their ugly heads once more. “It's probably one of the more striking findings from the paper, where you see these images and [the cancer cells] are actually in the process of leaving the scar,” Joyce added.
These results begged the question: Could scar formation be stopped? And if so, could recurrence be prevented? As the team soon discovered, this was the tricky part. First, they attempted to inhibit transforming growth factor beta (TGF-β) signaling, which had stood out to them as a key pathway driving fibrosis, in mice. There was no effect.
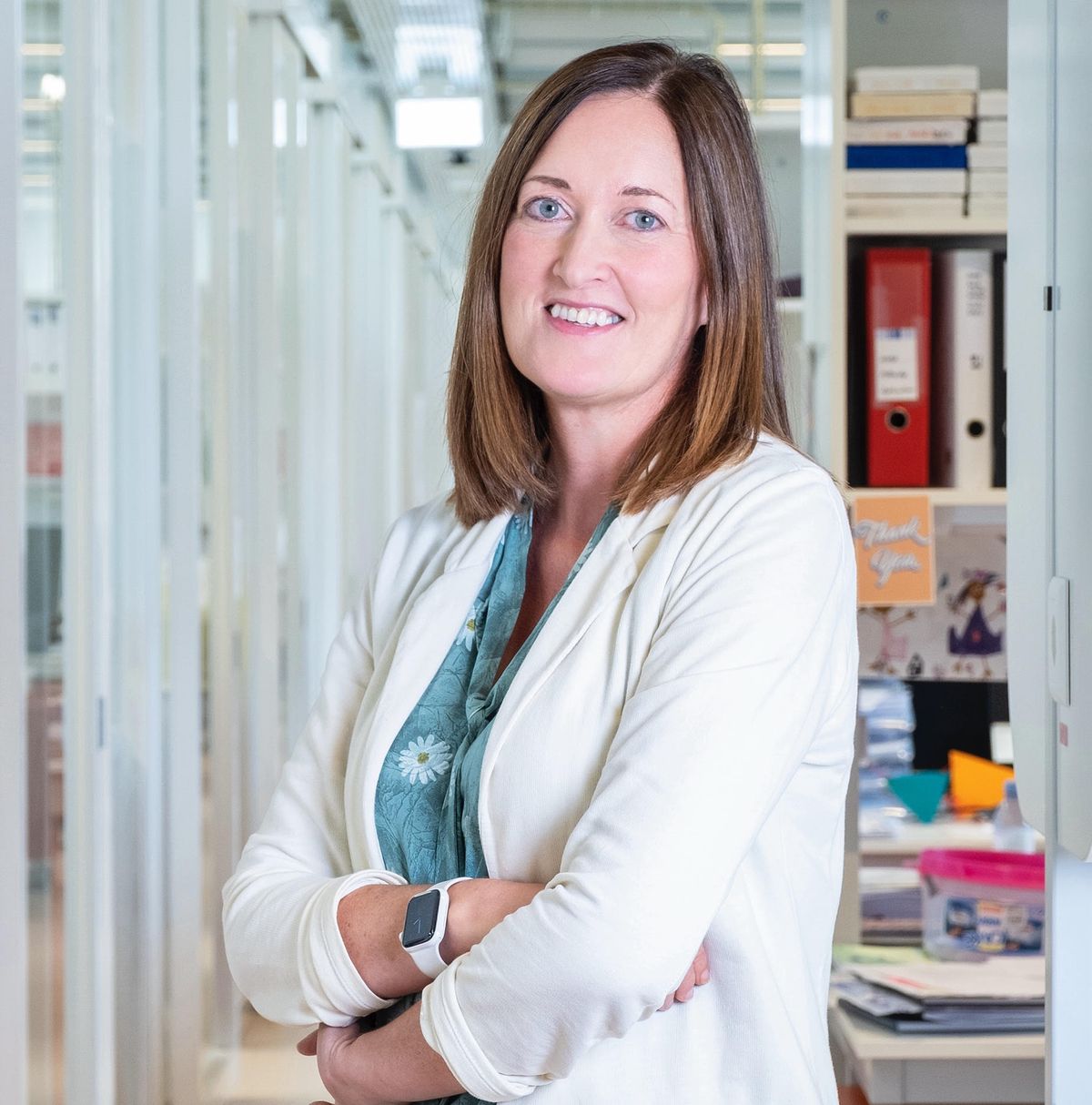
Next, they tried a broad-spectrum anti-inflammatory treatment to prevent neuroinflammation pathways, which had similarly been highlighted as a driver of fibrosis and scar formation. Again, they saw no effect. Even these two therapies in combination showed no reduction in scar formation or enhanced survival of the animals.
The breakthrough that they hoped for came when they tried inhibition of TGF- β signaling and anti-inflammatory treatment with the original macrophage-targeted therapy. “What we found is that these three drugs in combination essentially blocked the scars from forming,” Joyce said. In a long-term preclinical study, all but one mouse that received the treatment survived.
An intriguing aspect of GBM is that other treatments, including surgery, chemotherapy, and radiation, also commonly result in fibrotic scarring and tumor recurrence. One of the next steps for Joyce and her team is to explore the scars that arise from these other treatments to determine if the mechanisms of scar formation and tumor recurrence are the same and if they, too, can be prevented. The research “…could improve survival rates, which are currently very poor for glioblastoma patients,” Jenkins summarized.
References
- Quail DF, et al. The tumor microenvironment underlies acquired resistance to CSF-1R inhibition in gliomas. Science. 2016;352(6288):aad3018.
- Watson SS, et al. Fibrotic response to anti-CSF-1R therapy potentiates glioblastoma recurrence. Cancer Cell. 2024;42(9):1507-1527.e11.