ABOVE: Researchers reported a novel bacterial immune system that produces tailless, non-infectious phages. iStock, koto_feja
Microbiologists have studied bacterial defense mechanisms against phages for decades. But until less than a decade ago, there were only a few known bacterial immune systems: induced suicide mechanisms, restriction-modification, and CRISPR-Cas systems.1-3
In 2018, microbiologist Rotem Sorek and his colleagues at the Weizmann Institute revealed that bacterial antiphage defense mechanisms are much more diverse than originally thought.4 Since then, Sorek’s team and others have discovered a number of novel bacterial immune systems, including the Bil system (a primary component of the system is the Bil protein).5 Sorek next wanted to understand how the Bil system works.
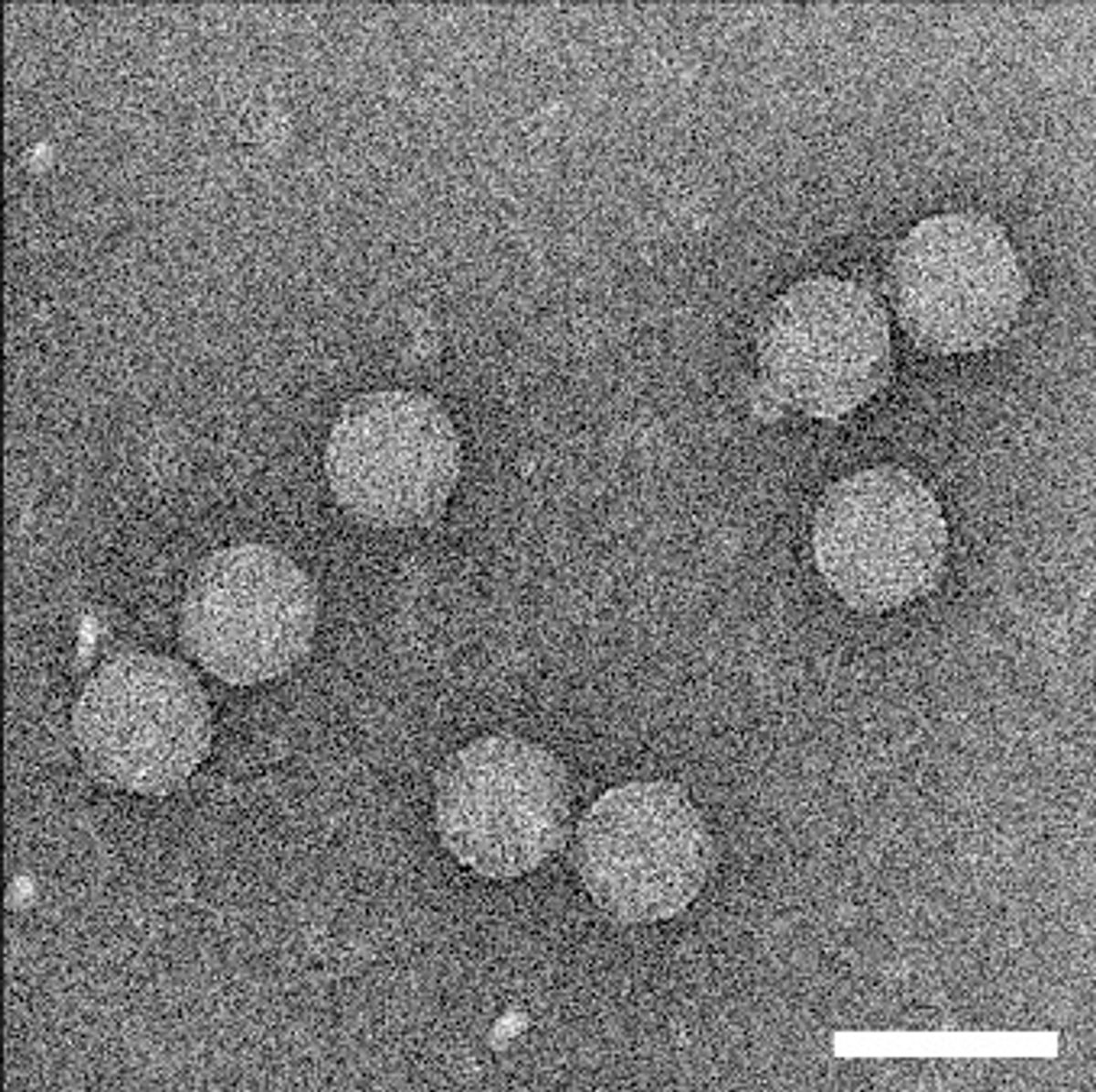
In a recent study in Nature, Sorek and his colleagues showed that bacteria can use this system to covalently attach the bacterial protein Bil to the spike protein of an infecting phage’s tail, which is crucial for the tail assembly of its progenies and the infection of new host cells.6 The researchers noted that the presence of Bil in phage-infected bacteria resulted in the production of phage progenies that were either tailless or fully assembled but less infectious, protecting the rest of the bacterial population.
The Bil system is mechanistically similar to ubiquitin pathways because it involves the covalent attachment of ubiquitin-like proteins (Ubl) by ubiquitin-conjugating enzymes.7 Sorek’s interest in the Bil protein first piqued because of its structural similarity to interferon-stimulated gene 15 (ISG15), a human Ubl, which is how it got its name (bacterial ISG15-like, or Bil for short).8 In humans, ISG15 is part of an innate antiviral defense pathway that’s known to protect against HIV and influenza, although its mechanism is not well-understood.
“Many of these bacterial systems have been shown to be the evolutionary origin of different human immune systems,” said microbiologist Jens Hör, a former postdoctoral fellow in Sorek’s lab and coauthor of the study. “We thought that if we figure out how it works in bacteria, we might also advance the human field a bit.”
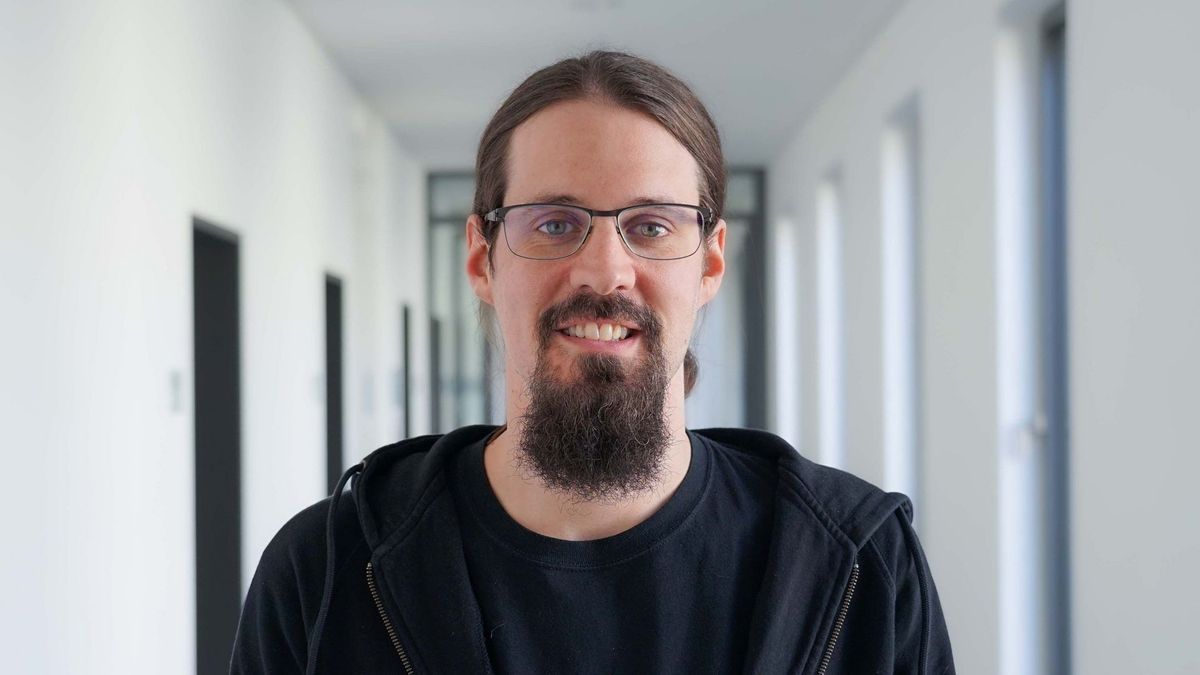
Kevin Corbett, a structural biologist at the University of California, San Diego who was not involved in the study, said that Bil seems to truly resemble the eukaryotic ubiquitination machinery. Corbett and his group concurrently published a paper on the structural and molecular details of the Bil system in the same issue of Nature.9
“This is the first time that bacteria have been shown to perform protein conjugation using machinery like this, particularly a ubiquitin-like protein,” said Corbett.
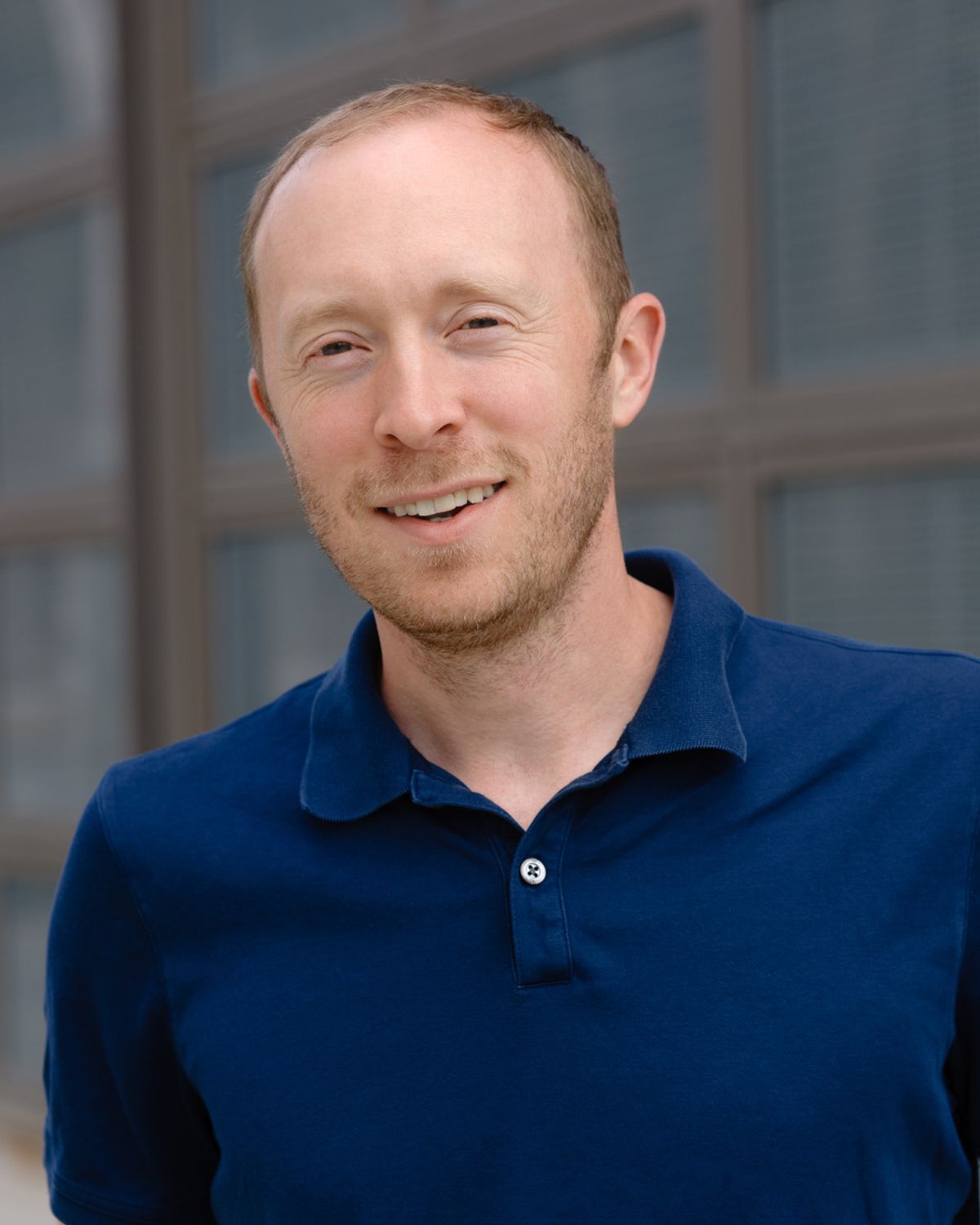
To understand how Bil works, Sorek’s team compared phage progeny from infected bacteria with and without the system. They performed density gradient centrifugation to separate phages from bacterial lysates and found that the bacteria that expressed Bil produced a higher density band in the gradient. When the scientists isolated the higher density band and viewed it under a transmission electron microscope, they were surprised by the sight of tailless phages. The team believes that the higher buoyant density could be explained by the higher DNA-to-protein ratio in tailless phages.
While not all the phage progenies end up tailless, Sorek and his team showed that even the fully assembled progenies showed a reduced capability of infecting other cells. By performing immunoprecipitation mass spectrometry, the authors showed that Bil is specifically conjugated to the spike protein at the tip of the phage’s tail. Hör said that Bil’s conjugation to the spike protein likely inhibits the interaction between the phage and its entry receptor on new host cells, which could explain why some of the tailed progenies are unable to infect.
Hör mentioned that some phages have enzymatic domains in their tails that could reverse Ubl conjugation reactions like the one performed by Bil. “One important question that we didn’t answer in our study is how phages can overcome bacterial defense by the Bil system,” he said.
- Lopatina A, et al. Abortive infection: Bacterial suicide as an antiviral immune strategy. Annu Rev Virol. 2020;7(1):371-384.
- Heitman J. On the origins, structures and functions of restriction-modification enzymes. Genet Eng (N Y). 1993;15:57-108.
- Hille F, Charpentier E. CRISPR-Cas: Biology, mechanisms and relevance. Philos Trans R Soc Lond B Biol Sci. 2016;371(1707):20150496.
- Doron S, et al. Systematic discovery of antiphage defense systems in the microbial pangenome. Science. 2018;359(6379):eaar4120.
- Millman A, et al. An expanded arsenal of immune systems that protect bacteria from phages. Cell Host Microbe. 2022;30(11):1556-1569.e5.
- Hör J, et al. Bacteria conjugate ubiquitin-like proteins to interfere with phage assembly. Nature. 2024;631(8022):850-856.
- Cappadocia L, Lima CD. Ubiquitin-like protein conjugation: Structures, chemistry, and mechanism. Chem Rev. 2018;118(3):889-918.
- Perng YC, Lenschow DJ. ISG15 in antiviral immunity and beyond. Nat Rev Microbiol. 2018;16(7):423-439.
- Chambers LR, et al. A eukaryotic-like ubiquitination system in bacterial antiviral defence. Nature. 2024;631(8022):843-849.