ABOVE: Using computer simulations, researchers discovered that under stress, bacterial colonies slow their growth to create space from their neighbors, forming a pattern of concentric circles. ©iStock, Scharvik
Under ideal conditions, bacterial cell proliferation is exponential, transforming an empty Petri dish into a lush “lawn” of growth. However, as a bacterial colony develops, “growing pains” are inevitable, as proliferating cells must balance growth rates with the stress of overcrowding and nutrient resource limitations. Other factors, such as variations in the timing of cell division or the size of the cells, further complicate this balance and make studying population dynamics challenging.
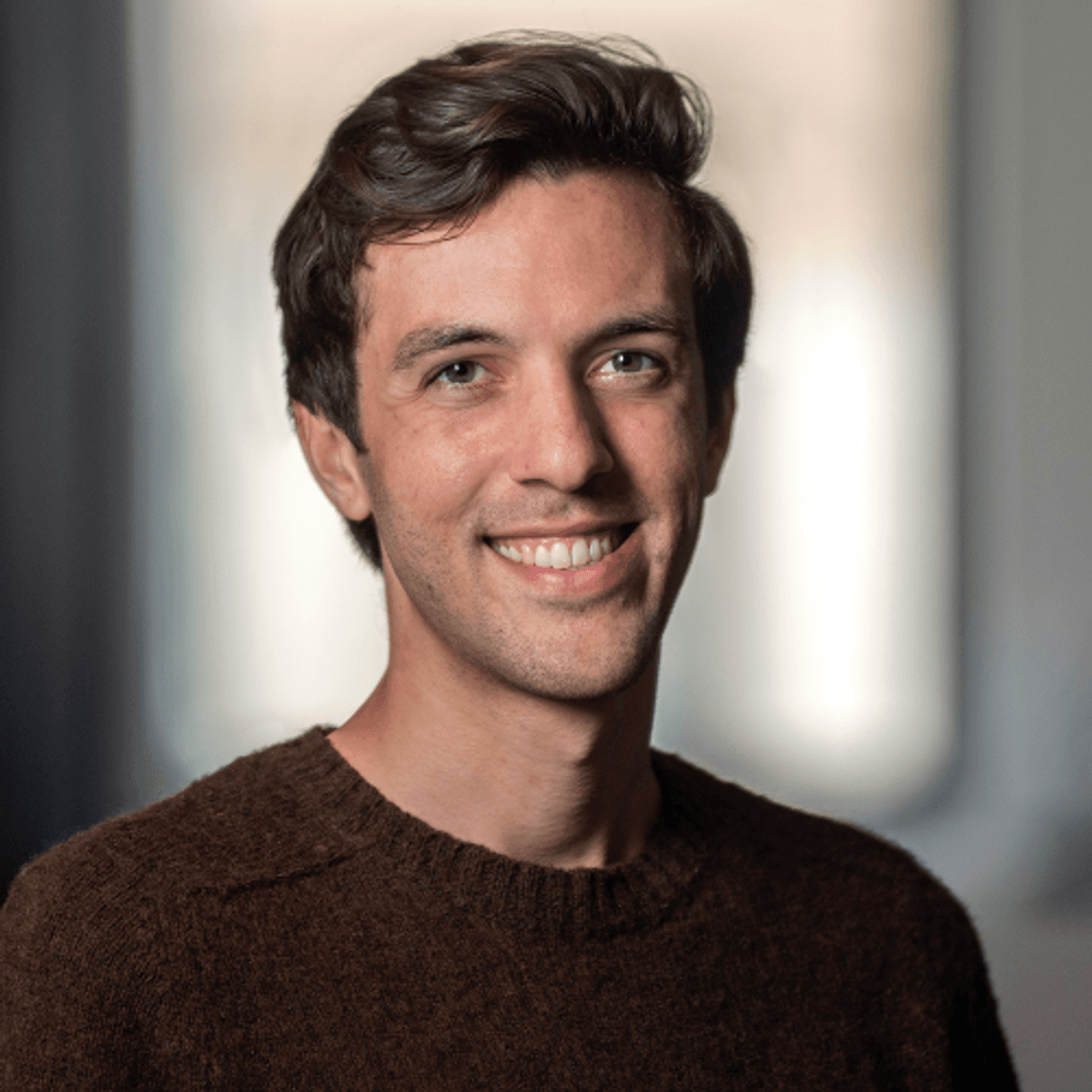
Instead of relying on traditional Petri plate experiments, researchers like Scott Weady, a computational biologist at the Flatiron Institute of the Simons Foundation, create simulations to model specific features of bacterial growth dynamics. Using these precisely controlled, artificial environments, Weady aimed to understand how cells respond to one of life’s universal challenges: stress.
In these simulations, Weady and his colleagues observed cell size and noticed an unexpected pattern that accompanied the colony’s expansion. “We start with a single cell, and it just starts growing. As it’s growing, they’re pushing and they expand into this big circle,” explained Weady. As the colony’s particles multiplied, they pushed against each other, intensifying cell crowding and slowing their proliferation rate. Their findings, published in Physical Review Letters, uncovered a stress-adapted growth strategy that could aid in modeling cellular responses to stressors and developing new strategies for controlling pathogen growth.1
First, Weady developed particle simulations, which modeled the proliferation behavior of a single particle—the computerized version of a bacterial cell—as it expanded into a relatively small colony. The artificial cell’s “birth length," as Weady described it, was about two micrometers by one micrometer and resembled a rod-shaped bacterium like Escherichia coli. The particles grew in proportion to their size, dividing once they reached twice their birth length.
However, as the colony grew outward from the center, a kind of cellular mosh pit erupted. “All of their neighbors are crowding around them, so [the cells] have to push a bunch of other cells out to grow, and then all of that’s happening while another neighbor is trying to push that other one out of the way,” said Weady.
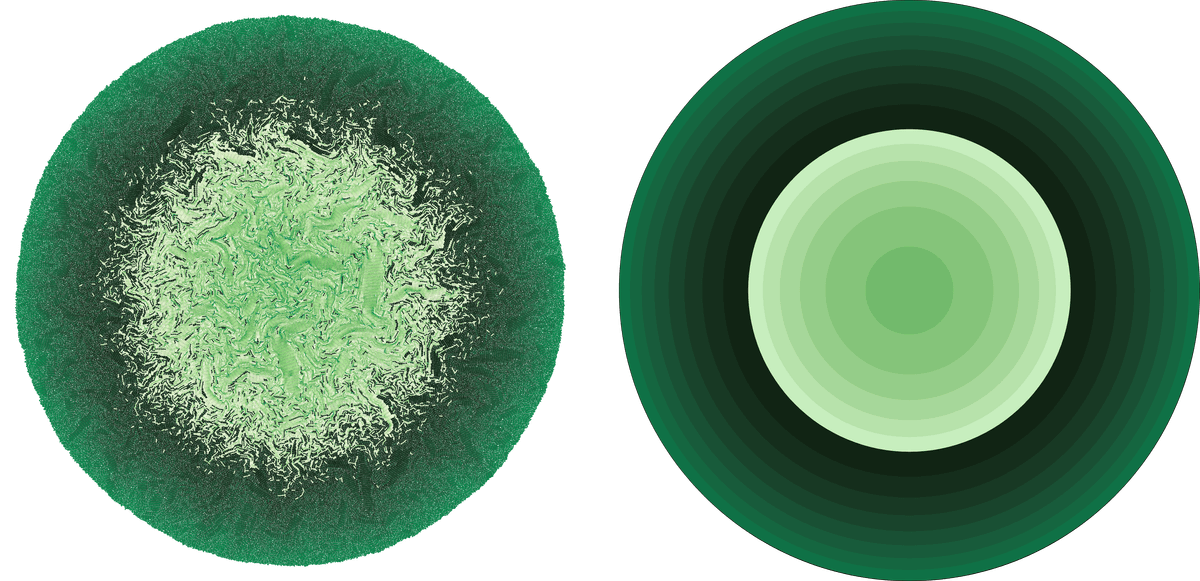
As the simulations went on, Weady noticed a pattern emerging. Their calculations revealed that, when push came to shove, the rapidly dividing bacteria at the colony’s center became a stress hub. Weady noted that as the experiment continued, new stress hubs emerged in their simulated Petri dish, leading to shorter cells–a sign of slowed growth. The particles formed concentric circles, reminiscent of tree rings, with each ring marking a cycle of growth, overcrowding, and slowed proliferation. More rings indicated more cycles, which reflected higher levels of stress from overcrowding. Cells at the edge of the colony (light green in the images) rapidly grew, encroaching on their neighbors’ space. Consequently, this slowed the growth of nearby cells (darker green in the images).
These initial particle simulations demonstrated how proliferation pans out with a relatively small number of cells. Based on this data, the researchers developed a continuum model to predict how the same process behaved with a much larger number of starting cells, ultimately reaching an identical final growth size. Weady saw that this model aligned closely with their particle simulations, reinforcing their observations that crowded bacterial cells stalled their growth in response to stress.
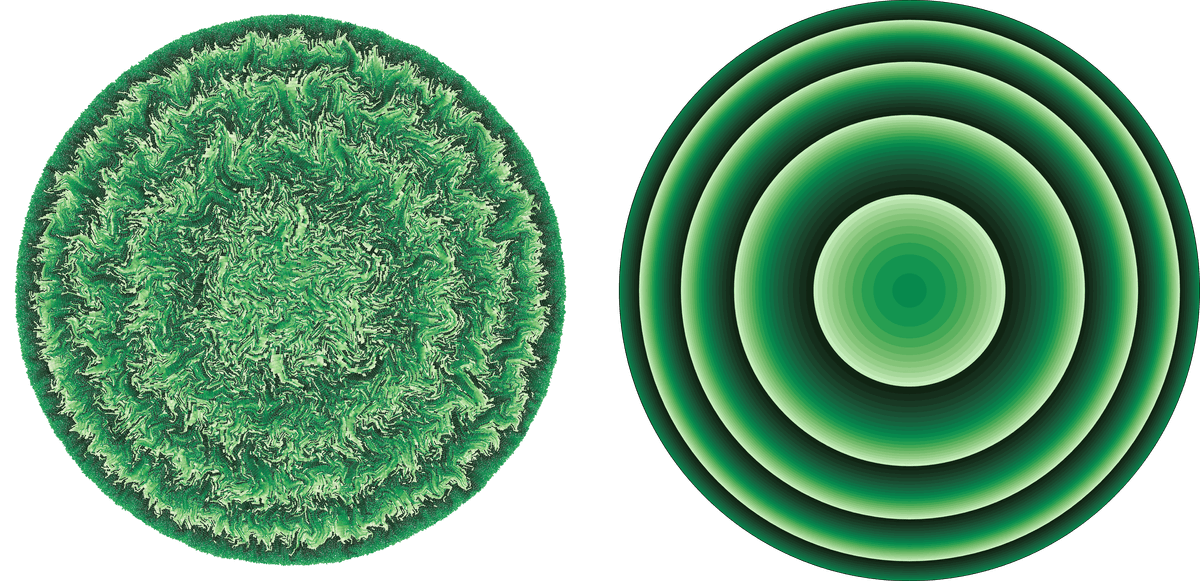
Understanding the fundamental biophysical processes of bacterial growth could help scientists use these insights to combat pathogenic bacteria during infections and improve microbial control. Weady sees potential in this modeling technology, which enables researchers to experiment with colony proliferation in various environments, such as on a fluid or a surface layer. “[Modeling these systems] is an exciting thing, because using these models can hopefully make a prediction that we can then go and test, versus the other way around.” He hopes to see similar results when applying this technology to real bacterial colonies.
- Weady S, et al. Mechanics and morphology of proliferating cell collectives with self-inhibiting growth. Phys Rev Lett. 2024;133(15):158402.