ABOVE: Conquerors of the animal kingdom, Wolbachia bacteria, infect the reproductive tissues of many different arthropods, including the wasp Nasonia vitripennis. Sarah Bordenstein
In the mid-1920s, entomologist Marshall Hertig and pathologist Simeon Burt Wolbach decided to step out of their laboratories at Harvard University and collect insects in the surroundings of Boston and Minneapolis. The duo studied the Rickettsiales order—a group of obligate intracellular bacteria that rely on host cells for replication. In the early 1900s, researchers undertook the task of characterizing bacterial members of this order in arthropods and studying the diseases they cause in humans, such as Rocky Mountain spotted fever and typhus. Hertig and Wolbach wanted to determine the frequency of these Rickettsia-like organisms in insects and identify characteristics that would enable their distinction from cell granules and artifacts. Among the specimens they brought back to the lab were over 20 Culex pipiens mosquitoes. By carefully dissecting the insect’s tissues and examining them under the microscope, the researchers identified for the first time tiny Rickettsia-like microorganisms in the mosquito’s ovaries, testes, and eggs.1
Wolbachia is endlessly fascinating.
—William Sullivan, University of California, Santa Cruz
A few years after their discovery, Hertig named these intracellular microorganisms Wolbachia pipientis. Since Hertig’s and Wolbach’s initial description in 1924, researchers around the world have delved into the biology of Wolbachia. They not only discovered the wide range of arthropods and nematodes that harbor the bacteria inside their cells, but also a surprising constellation of parasitic and mutualistic adaptations that make this bacterial endosymbiont an unmistakable example of evolutionary success.2,3
How Wolbachia Propagates: An Endosymbiont’s Dance for Vertical Transmission
Hertig’s and Wolbach’s early observations of Wolbachia in the eggs of C. pipiens strongly suggested that the bacteria were maternally transmitted. William Sullivan, a cell biologist at the University of California, Santa Cruz, was intrigued by how the endosymbiont propagates to the next generation. “Wolbachia is endlessly fascinating,” he said.
Sullivan learned from the research of Michael Turelli, an evolutionary biologist at the University of California, Davis, that the bacteria were spreading in fruit flies in California. So, he took his students on a field trip to investigate whether Wolbachia had reached the wineries in Santa Cruz County. According to Sullivan, it was through this activity and the students’ presentations and reports that he immersed himself in the world of the endosymbiont.
As Wolbachia are efficiently transmitted from the female host to her offspring, Sullivan wondered how the endosymbiont does that. Previous evidence suggested that some intracellular microbes use components of the cell cytoskeleton to be able to move within and between cells. This was the case of Listeria monocytogenes, a pathogenic bacteria known for causing listeriosis.4 In the early 1990s, researchers showed that L. monocytogenes hijacks the host’s cytoskeleton to form actin-rich structures called rocket tails, which enable the bacteria to zip around the cell cytoplasm.5
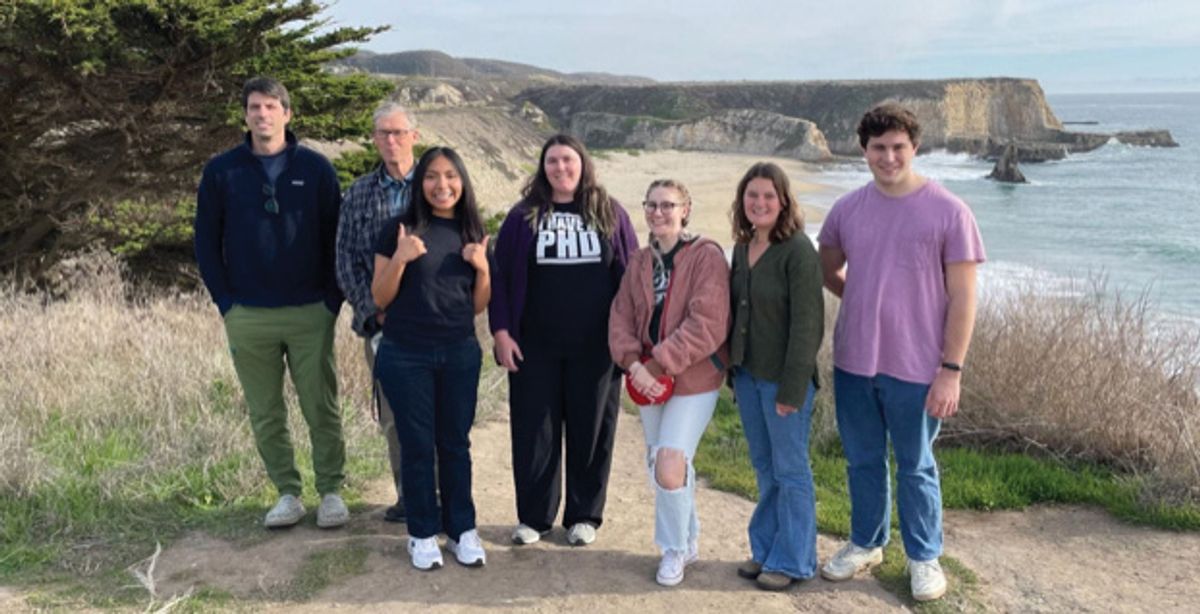
To explore if Wolbachia would also snatch components of the cytoskeleton to ensure its high transmission rates, Sullivan and his team first focused on the early stages of oogenesis. In the ovaries of Wolbachia-infected Drosophila melanogaster fruit flies, they found that the bacteria clearly localized at the anterior end of the oocyte and used the host’s microtubule network and the associated motor protein dynein to reach that subcellular localization.6
During late stages of egg development, Wolbachia concentrate at the posterior end of the mature oocyte, where germline precursor cells are located.7 To investigate whether microtubules played a role in the bacteria’s anterior to posterior reallocation, Sullivan’s team looked at the ovaries of Wolbachia-infected fruit flies in the late stages of oogenesis. Microtubules undergo a major rearrangement inside the oocyte during the mid-stages of oogenesis, shifting their polarity so that the microtubules plus ends concentrate at the posterior pole of the oocyte.8 Therefore, Sullivan and his team focused on the motor protein kinesin, which is known for moving towards microtubules plus ends. Using mutant flies that lacked kinesin, they found that Wolbachia hijacked this motor protein to reallocate to the posterior end and place themselves at the site of germline cell formation, likely ensuring the bacterial vertical transmission.9 “That means Wolbachia has to be really sophisticated,” Sullivan explained. “There must be some surface proteins early in oogenesis to engage the motor [protein] dynein and then [Wolbachia] must turn off those surface proteins and turn on a surface protein that engages kinesin.”
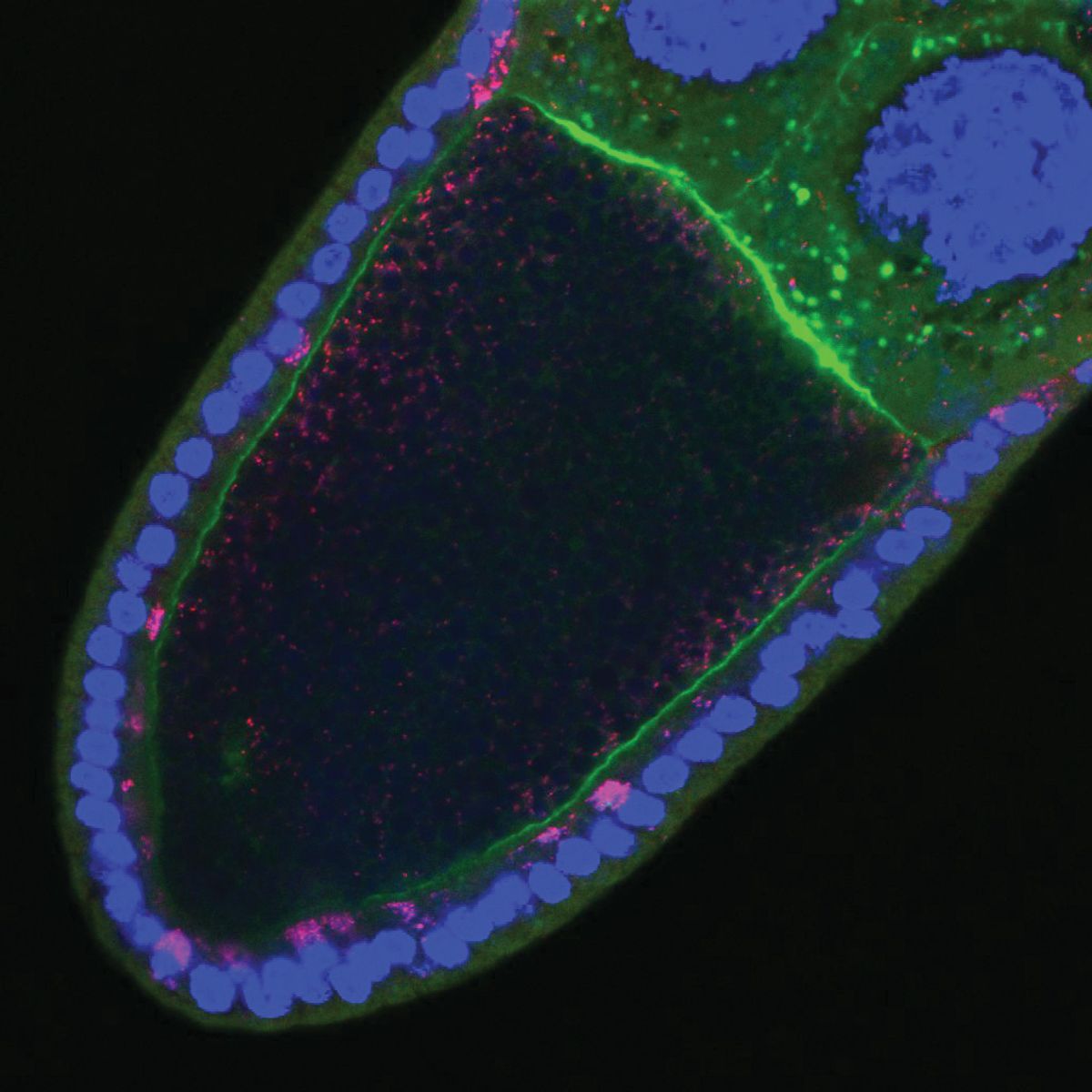
Because reaching the posterior pole of the mature oocyte is a milestone for the vertical transmission of Wolbachia, Sullivan investigated the bacteria-kinesin association more closely. Kinesins are tetramers composed of two heavy chains (KHC), responsible for motor activity, and two light chains (KLC), which can bind cargo and act as a linker protein.10 By knocking down KLC, Sullivan’s team found that the bacteria were not only able to reach the posterior pole but their numbers also increased drastically.11 “This was just one of those crazy results,” he recalled. Overexpression of KHC induced a similar increase in Wolbachia levels at that oocyte subcellular location, suggesting that KHC availability limits the bacteria transport. Since the oocyte has a limited number of kinesins to move essential components to the posterior pole, Wolbachia must be careful not to disrupt this process, Sullivan explained. “It needs that germline to be intact for itself. So, it has evolved to be this really weak competitor,” he added.
While the endosymbiont dynamic choreography inside the oocyte was captivating to Sullivan, it was not the only Wolbachia-related phenomenon that piqued his interest. “I got really excited about cytoplasmic incompatibility,” he said.
Wolbachia Bacteria and the Art of Reproductive Manipulation
Among the reproductive manipulations associated with Wolbachia, cytoplasmic incompatibility (CI) is probably one of the most enigmatic. First identified by biologist Hannes Laven in the 1950s, CI designates a phenomenon in which breeding between insects of the same species fails to produce a viable offspring. Laven observed this peculiar mating incompatibility while studying C. pipiens populations from different geographical locations. Based on his experiments, he proposed that an incompatibility factor present in the cytoplasm was passed from the parents to the progeny.12 Laven was also one of the first to recognize that CI could contribute to speciation and mosquito population control.13,14
It was only in the early 1970s that researchers established the link between CI and Wolbachia when they observed significant numbers of the microorganism in the eggs and embryos of incompatible crosses of C. pipiens.15
According to Sullivan, CI stands out as Wolbachia’s cleverest reproductive manipulation because it requires a collaboration between males and females. “If a male is infected but not a female, the eggs don’t hatch. But if a male is infected as well as the female, the eggs hatch. So, if you’re an uninfected female in an infected population, you’re at a disadvantage,” he explained.
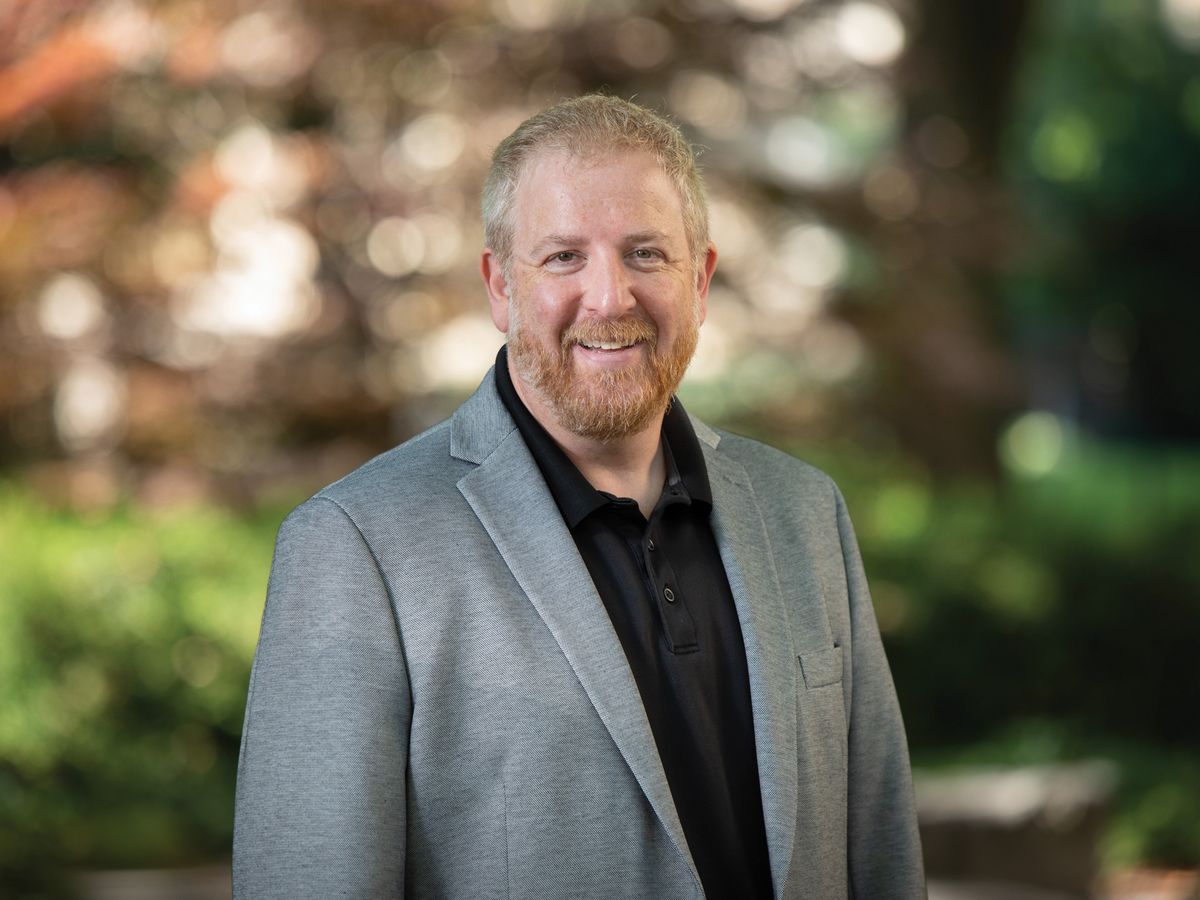
Sullivan and others were intrigued by how Wolbachia tinkered with the reproductive cells of insects to induce CI, and he approached this reproductive puzzle from a chromosome perspective. Early studies from the 1990s suggested that Wolbachia disrupted paternal chromosome dynamics during fertilization and early embryonic development, and scientists hypothesized that the bacteria caused a mismatch between the timing of events taking place at the beginning of embryogenesis.16,17
To test this idea, Sullivan and his team developed a cytological technique to study the fertilization and first mitotic division in living embryos of the wasp Nasonia vitripennis. They observed that the male pronuclei in CI embryos showed delayed nuclear envelope breakdown—a signal of entry into mitosis—causing asynchrony between the male and female pronuclei during the early stages of embryonic development.18 In a subsequent study, the team found that CI affected chromatin condensation and proper DNA replication in the male pronucleus by delaying the deposition of key histones and prolonging the retention of the proliferating cell nuclear antigen, both of which could contribute to mitotic defects and embryo lethality.19
A Phage’s Gift
While Sullivan and other researchers were exploring Wolbachia’s reproductive manipulations at the cellular level, Seth Bordenstein, a biologist at Pennsylvania State University, wanted to understand the genetic secrets that enabled this endosymbiont to command its host. “When I learned about [Wolbachia], I said, ‘how is it possible that a simple microorganism with a small genome can live inside an animal host and do these amazing reproductive modifications in order to benefit itself?’ It felt like Wolbachia was a puppeteer of a very complex host,” Bordenstein said.
Phage WO holds a lot of the powers that Wolbachia is so well known for.
—Seth Bordenstein, Pennsylvania State University
Endosymbionts were thought to lose a significant amount of their genomes when they entered an obligate symbiotic relationship with their hosts—a process termed genome reduction.20 Researchers considered that mobile genetic elements, such as bacteriophages and transposons, were the first to be eliminated in this process; yet, the sequencing of Wolbachia’s genome revealed otherwise, indicating that the bacteriophage-like particles first identified in the endosymbiont in the late 1970s incorporated their genetic materials into the streamlined microbes.21-23
Phages were known to grant adaptive traits to free-living bacteria, so Bordenstein wondered if the bacteriophage found in Wolbachia, phage WO, would do the same for the endosymbiont and affect its ability to cause CI.
Bordenstein’s team compared the genome of Wolbachia strains known to cause CI with one that did not cause the mating incompatibility and found that only two genes were shared between CI-causing strains.24 “Nobody finds two genes in a multiomics analysis, they usually find hundreds of genes. So, we thought we were either dead wrong or dead right,” Bordenstein recalled.
Since Wolbachia do not replicate outside of the cells of their hosts, and scientists cannot culture them in the lab, Bordenstein and his team created transgenic fruit flies that expressed the two genes the team named CI factors A (cifA) and B (cifB). The individual expression of the genes did not recreate the CI phenotype. That’s when they decided to express cifA and cifB together. “It was our last-ditch effort, and we were able to find that the two genes expressed together from a fly genome recapitulated the cytoplasmic incompatibility sperm trait,” Bordenstein said. In the same year that these findings were made public, researchers led by Yale University biologist Mark Hochstrasser reported similar results in a different Wolbachia strain, collectively providing the first evidence for the genetic basis of Wolbachia’s CI.25
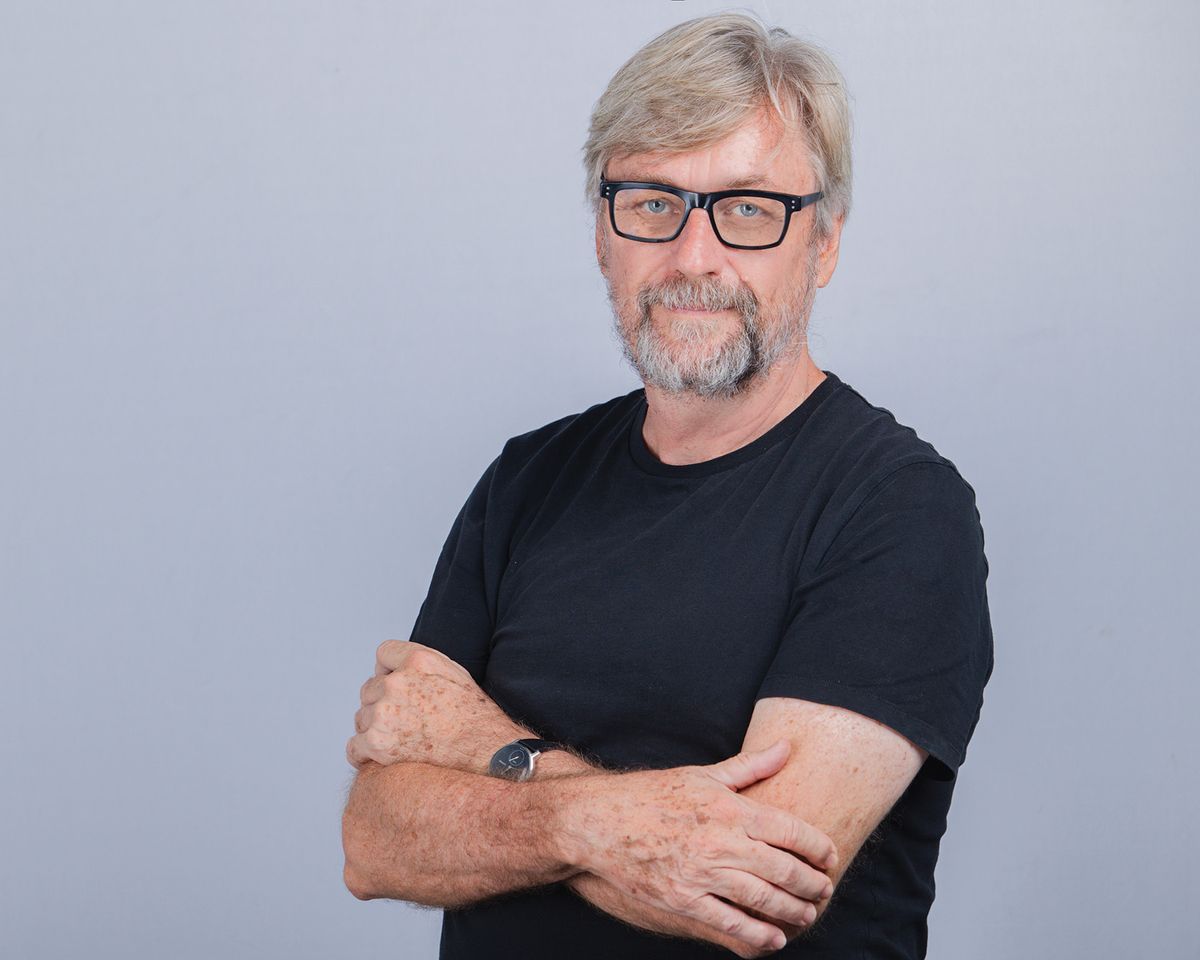
Bordenstein and his team went on to explore the effects of cifA and cifB by assessing whether the genes could contribute to CI rescue seen when infected males mate with infected females. Using transgenic fruit flies expressing these genes, they found that female expression of cifA rescued CI and repealed the Wolbachia-induced embryonic death.26
The ability to cause, and in females rescue, CI was not the only secret kept in the phage WO genome. Bordenstein knew that Wolbachia could shift the sex ratios in a population through a phenomenon termed male killing, and researchers considered this cunning strategy as another example of the endosymbiont’s abilities to ensure its presence in a large proportion of females in a host population.27 To investigate whether the phage WO genes were also linked to this phenotype, Bordenstein’s team used a comparative genomic approach and found a candidate gene they named WO-mediated killing (wmk).28 When the researchers transgenically expressed wmk in fruit flies, they found that wmk induced cytological defects and male embryonic death. “Phage WO holds a lot of the powers that Wolbachia is so well known for,” Bordenstein said.
Wolbachia Turn into a Microbial Ally in the Fight Against Diseases
Biologist Scott O’Neill has also been fascinated by Wolbachia’s biological tricks. The founder and chief executive officer of the World Mosquito Program first learned about the endosymbiont during his graduate studies. The wide range of phenotypic outcomes that the bacteria induced in arthropods, including CI and male killing, inspired O’Neill to explore Wolbachia as an agent for disease control.
Most mosquito-borne pathogens experience a significant period of development inside their vectors before they are transmitted to a new host, suggesting that the survival of the mosquitoes is of utmost importance for pathogen transmission. In the late 1990s, scientists identified a Wolbachia strain that shortened the lifespan of its natural D. melanogaster host.29 O’Neill saw this as a potential strategy to skew the age distribution of mosquito populations, thus impacting the vector’s ability to spread the pathogens they carry.
To explore Wolbachia’s potential mosquito-killing skills, O’Neill and his team first had to determine whether the life-shortening strain would adapt to a new host. They transferred the fruit fly’s native bacteria to a cell line derived from the mosquito Aedes albopictus and maintained this cell line for more than 200 serial passages before infecting other mosquito cell lines.30 When the team reintroduced the Wolbachia strain into the original host, they observed diminished life-shortening and CI capabilities compared to the native fruit fly bacteria, suggesting that the strain had adapted to its new mosquito host. The researchers then microinjected the mosquito-adapted life-shortening Wolbachia in uninfected embryos to generate mosquito lines carrying the endosymbiont. “Those transfer experiments were very difficult to do, and we spent a long time doing that work until we were successful,” O’Neill recalled. Then, the team zoomed in on Aedes aegypti, a major vector of epidemiologically relevant viruses, paying particular attention to the female mosquitoes, which are blood feeders that can transmit disease-causing pathogens as they search for their next blood meal.31 The life-shortening Wolbachia strain not only reduced the longevity of female A. aegypti mosquitoes by approximately 50 percent, but it also induced a strong CI phenotype and was maternally transmitted to the offspring.32
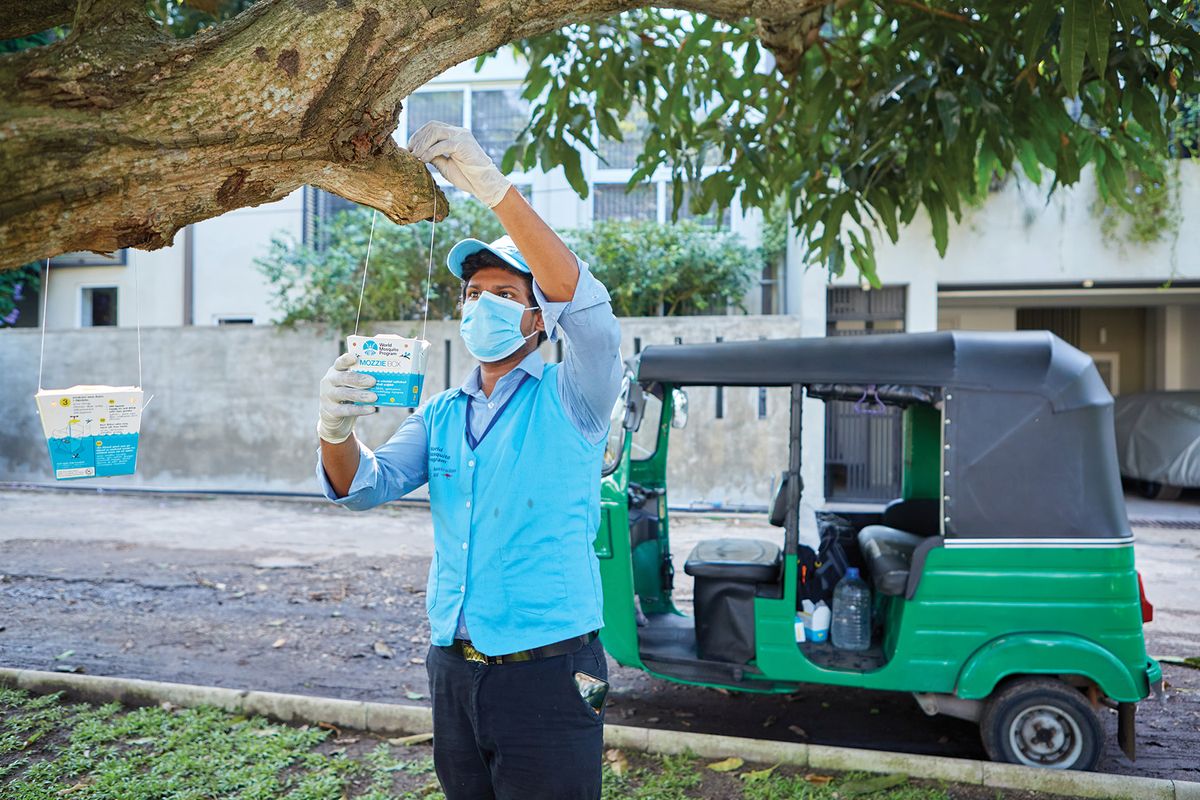
While disrupting mosquito biology seemed a promising strategy for controlling mosquito-borne pathogens, Wolbachia still had another trick up its sleeves. In the mid-2000s, O’Neill collaborated with Karyn Johnson, a molecular virologist at the University of Queensland, who was interested in studying the effects of the endosymbiont on the viruses carried by D. melanogaster. The researchers examined the survival rates of flies infected or not with viral pathogens and found that Wolbachia-carrying fruit flies had a delayed mortality that corresponded to a delayed accumulation of the viruses in the insects.33 “That result was very striking and very powerful,” O’Neill said.
O’Neill realized that this was yet another ability of Wolbachia that could be leveraged to control insect-borne diseases, particularly those transmitted by mosquitoes. Using the Wolbachia-infected A. aegypti mosquitoes that they previously generated, O’Neill and his team showed that Wolbachia-carrying mosquitoes had reduced loads of dengue and chikungunya viruses, suggesting that the endosymbiont blocked these viruses from replicating inside their natural vector.34
Over the past 15 years, O’Neill and others have translated these early laboratory findings into field strategies to control mosquito-borne diseases and shown encouraging results in reducing dengue transmission in many areas of the world.35
“It’s often undervalued by politicians and by skeptics of science that our investments in fundamental basic research can transition into something grand,” said Bordenstein. “A lot of Wolbachia biology was basic science initially. And now, it’s used to curb diseases transmitted by mosquitoes and diseases caused by filarial nematodes,” he noted. “We’re talking about millions of people that could be having their burden of disease or risk of disease reduced from this basic science.”
A Bright Future for a Centenarian Microbe
While researchers have uncovered many of Wolbachia’s biological enigmas over the past 100 years, puzzles remain to be solved. According to Bordenstein and Sullivan, one such puzzle is the mechanism that mediates CI rescue. Bordenstein plans to tackle this phenomenon to better understand how Wolbachia-infected females de-encrypt the changes the endosymbiont induces in males, allowing embryonic development to occur.
Understanding how Wolbachia affects somatic cells is another interesting area, according to Sullivan. “Wolbachia has this huge somatic life,” he said. His team is investigating this facet of the endosymbiont’s life by exploring how Wolbachia can influence its host’s behavior. “That’s a whole amazing story of Wolbachia targeting different regions of the brain to affect behavior,” Sullivan said.
How microorganisms, including Wolbachia, might contribute to species formation is another area of curiosity for Bordenstein. “Microbes were essentially ignored from the last 100 years of speciation biology, which had largely been dominated since the modern synthesis by genetics and finding the number and types of genes,” he explained. Over the years, researchers have found evidence of how microbial symbionts can influence the origin of species.36 “Once we can open this Pandora’s box a little [more], we’ll really start to know whether symbiont microbes are just as important as nuclear genes are for speciation,” Bordenstein said. “That’s a big question.”
- Hertig M, Wolbach SB. Studies on Rickettsia-like micro-organisms in insects. J Med Res. 1924;44(3):329-374.7.
- Weinert LA, et al. The incidence of bacterial endosymbionts in terrestrial arthropods. Proc Biol Sci. 2015;282(1807):20150249.
- Lefoulon E, et al. Breakdown of coevolution between symbiotic bacteria Wolbachia and their filarial hosts. PeerJ. 2016;4:e1840.
- CDC. Listeria (Listeriosis). Centers for Disease Control and Prevention. Last Reviewed July 19, 2024. Accessed July 22, 2024.
- Dabiri GA, et al. Listeria monocytogenes moves rapidly through the host-cell cytoplasm by inducing directional actin assembly. Proc Natl Acad Sci USA. 1990;87(16):6068-6072.
- Ferree PM, et al. Wolbachia utilizes host microtubules and Dynein for anterior localization in the Drosophila oocyte. PLoS Pathog. 2005;1(2):e14.
- Hadfield SJ, Axton JM. Germ cells colonized by endosymbiotic bacteria. Nature. 1999;402(6761):482.
- Steinhauer J, Kalderon D. Microtubule polarity and axis formation in the Drosophila oocyte. Dev Dyn. 2006;235(6):1455-1468.
- Serbus LR, Sullivan W. A cellular basis for Wolbachia recruitment to the host germline. PLoS Pathog. 2007;3(12):e190.
- Marx A, et al. Structures of kinesin motor proteins. Cell Motil Cytoskeleton. 2009;66(11):958-966.
- Russell SL, et al. Wolbachia and host germline components compete for kinesin-mediated transport to the posterior pole of the Drosophila oocyte. PLoS Pathog. 2018;14(8):e1007216.
- Laven H. Cytoplasmic inheritance in Culex. Nature. 1956;177(4499):141-142.
- Laven H. Speciation by cytoplasmic isolation in the Culex pipiens-complex. Cold Spring Harb Symp Quant Biol. 1959;24:166-173.
- Laven H. Eradication of Culex pipiens fatigans through cytoplasmic incompatibility. Nature. 1967;216(5113):383-384.
- Yen JH, Barr AR. New hypothesis of the cause of cytoplasmic incompatibility in Culex pipiens L. Nature. 1971;232(5313):657-658.
- Callaini G, et al. Wolbachia-induced delay of paternal chromatin condensation does not prevent maternal chromosomes from entering anaphase in incompatible crosses of Drosophila simulans. J Cell Sci. 1997;110 (2):271-280.
- Lassy CW, Karr TL. Cytological analysis of fertilization and early embryonic development in incompatible crosses of Drosophila simulans. Mech Dev. 1996;57(1):47-58.
- Tram U, Sullivan W. Role of delayed nuclear envelope breakdown and mitosis in Wolbachia-induced cytoplasmic incompatibility. Science. 2002;296(5570):1124-1126.
- Landmann F, et al. Wolbachia-mediated cytoplasmic incompatibility is associated with impaired histone deposition in the male pronucleus. PLoS Pathog. 2009;5(3):e1000343.
- McCutcheon JP, et al. How do bacterial endosymbionts work with so few genes?. PLoS Biol. 2024;22(4):e3002577.
- Wu M, Set al. Phylogenomics of the reproductive parasite Wolbachia pipientis wMel: A streamlined genome overrun by mobile genetic elements. PLoS Biol. 2004;2(3):E69.
- Wright JD, et al. The ultrastructure of the rickettsia-like microorganism Wolbachia pipientis and associated virus-like bodies in the mosquito Culex pipiens. J Ultrastruct Res. 1978;63(1):79-85.
- Masui S, et al. Bacteriophage WO and virus-like particles in Wolbachia, an endosymbiont of arthropods. Biochem Biophys Res Commun. 2001;283(5):1099-1104.
- LePage DP, et al. Prophage WO genes recapitulate and enhance Wolbachia-induced cytoplasmic incompatibility. Nature. 2017;543(7644):243-247.
- Beckmann JF, et al. A Wolbachia deubiquitylating enzyme induces cytoplasmic incompatibility. Nat Microbiol. 2017;2:17007.
- Shropshire JD, et al. One prophage WO gene rescues cytoplasmic incompatibility in Drosophila melanogaster. Proc Natl Acad Sci USA. 2018;115(19):4987-4991.
- Hurst GDD, et al. Male-killing Wolbachia in two species of insect. Proc Biol Sci. 1999;266(1420):735.
- Perlmutter JI, et al. The phage gene wmk is a candidate for male killing by a bacterial endosymbiont. PLoS Pathog. 2019;15(9):e1007936.
- Min KT, Benzer S. Wolbachia, normally a symbiont of Drosophila, can be virulent, causing degeneration and early death. Proc Natl Acad Sci USA. 1997;94(20):10792-10796.
- McMeniman CJ, et al. Host adaptation of a Wolbachia strain after long-term serial passage in mosquito cell lines. Appl Environ Microbiol. 2008;74(22):6963-6969.
- WHO. Vector-borne diseases. World Health Organization. Published March 2, 2020. Accessed July 30, 2024.
- McMeniman CJ, et al. Stable introduction of a life-shortening Wolbachia infection into the mosquito Aedes aegypti. Science. 2009;323(5910):141-144.
- Hedges LM, et al. Wolbachia and virus protection in insects. Science. 2008;322(5902):702.
- Moreira LA, et al. A Wolbachia symbiont in Aedes aegypti limits infection with dengue, Chikungunya, and Plasmodium. Cell. 2009;139(7):1268-1278.
- Caragata EP, et al. Wolbachia as translational science: Controlling mosquito-borne pathogens. Trends Parasitol. 2021;37(12):1050-1067.
- Shropshire JD, Bordenstein SR. Speciation by symbiosis: The microbiome and behavior. mBio. 2016;7(2):e01785.