ABOVE: The lac operon indicated that genes could be organized and activated under specific conditions. © istock.com, selvanegra
In 1947, Jacques Monod, a biochemist at the Pasteur Institute, observed that Escherichia coli metabolized lactose only if glucose, the bacteria’s preferred sugar source, was absent.1 He began studying the system by investigating the lactose-digesting enzyme, β-galactosidase.2 Although he identified various substrates of the enzyme, the regulatory mechanism that controlled the metabolic switch remained elusive.3
When biologist François Jacob joined the institute in 1950, his research on how lambda bacteriophages integrated their genome into E. coli couldn’t seem more unrelated to Monod’s enzyme studies.4 Jacob used conjugation, the transfer of nucleic acid between bacteria through a bridge-like connection, to study how phage-infected E. coli developed resistance to secondary phage infections; Jacob and others interrupted this process to investigate the transfer of the phage genetic material and order of genes.5
It provides a way to leap off and say, 'how can we use this information to develop new, modern switches that can be used in eukaryotic systems and mammalian systems.'
—Mitchell Lewis, University of Pennsylvania
Jacob and Monod realized that they could use a similar approach to transfer genes between normal bacteria and mutants that either always expressed or entirely lacked β-galactosidase. Based on these experiments, the two scientists demonstrated in 1959 that an inhibitory gene product controlled the expression of β-galactosidase.6 This inhibition was inducible, ensuring that the bacteria only produced the lactose-digesting enzyme when lactose was available to digest. While scientists had previously understood that genes contained the instructions for building all of the cell’s necessary proteins, this provided one of the first examples of gene regulation by repression in response to changes in the environment.7
Jacob drew a parallel between the findings of β-galactosidase induction and bacteriophage production; both appeared to be controlled by some regulatory mechanism that repressed expression of the genes. Using lactose metabolism mutants, Jacob and Monod determined that the repressing element bound to a region of DNA, which they called the operator, and that this controlled the expression of a cluster of genes, which they called the lac operon, that was responsible for lactose digestion.8
“Prior to that work, the working model had been that the β-galactosidase, the enzyme produced by the lac operon, was there all the time,” said Stephen Busby, a biologist at the University of Birmingham. “[Scientists thought] it was made there all the time in the cell, but its activity was regulated.” Jacob and Monod’s discovery of a genetic mechanism of β-galactosidase expression would set the stage for unraveling the mechanisms of gene regulation.
The lac Operon Explained
In 1964, Jacob and Monod identified the promoter region for RNA polymerase.9 A few years later, another team mapped the genes of the lac operon—the repressor, promoter, operon, β-galactosidase, and two more structural genes, lactose permease and a transacetylase.10
While Jacob, Monod, and others previously showed that lactose metabolites containing galactose residues activated the lac operon, the true inducer remained elusive.11–13 Then, in 1972, a team identified allolactose, a lactose metabolite, as the lac operon inducer.14 In the presence of lactose, residual β-galactosidase produces allolactose, which binds to the repressor to release it from the DNA. The lac operon genes are expressed to digest the available lactose until this sugar is depleted, at which point the operon shuts down.
However, the lac operon was far from solved. “[It] turned out that there was a class of gene regulatory proteins that weren't repressors, [instead,] they were activators,” Busby said. Low cellular glucose leads to the production of cyclic adenosine monophosphate (cAMP); this molecule in turn binds to the cAMP receptor protein (CRP). This complex binds the lac operon, stabilizing the RNA polymerase to improve transcription of the lac operon genes when the repressor lifts.15,16
Another surprise came when scientists discovered that the operon had two promoters.17 Both drove lac operon gene expression if lactose was present, but one was specialized for induction under low glucose conditions. When CRP binds to stabilize the RNA polymerase, it also blocks one of these promoters; the available sequence is a stronger promoter, increasing gene expression and the production of lactose-digesting machinery in low-energy conditions compared to that from the second promoter.18–20
“That always struck me as really, really exciting to understand the magic finger that somehow can pick out one gene and say, ‘Gee, you're going to be turned on now,’” Busby said, who studied the role of cAMP/CRP in the recruitment of RNA polymerase in the lactose and other operons.
Walter Gilbert and Benno Müller-Hill answered another question about the lac operon when they isolated a protein, later named LacI, that was responsible for repressing the system, confirming previous indications that the repressor was a protein.21,22 Later, Gilbert and his colleague Allan Maxam showed that LacI was a tetrameric protein with four potential binding sites; they showed that two likely bound the operator sequencing, but the purpose behind the second set of subunits remained unclear.23
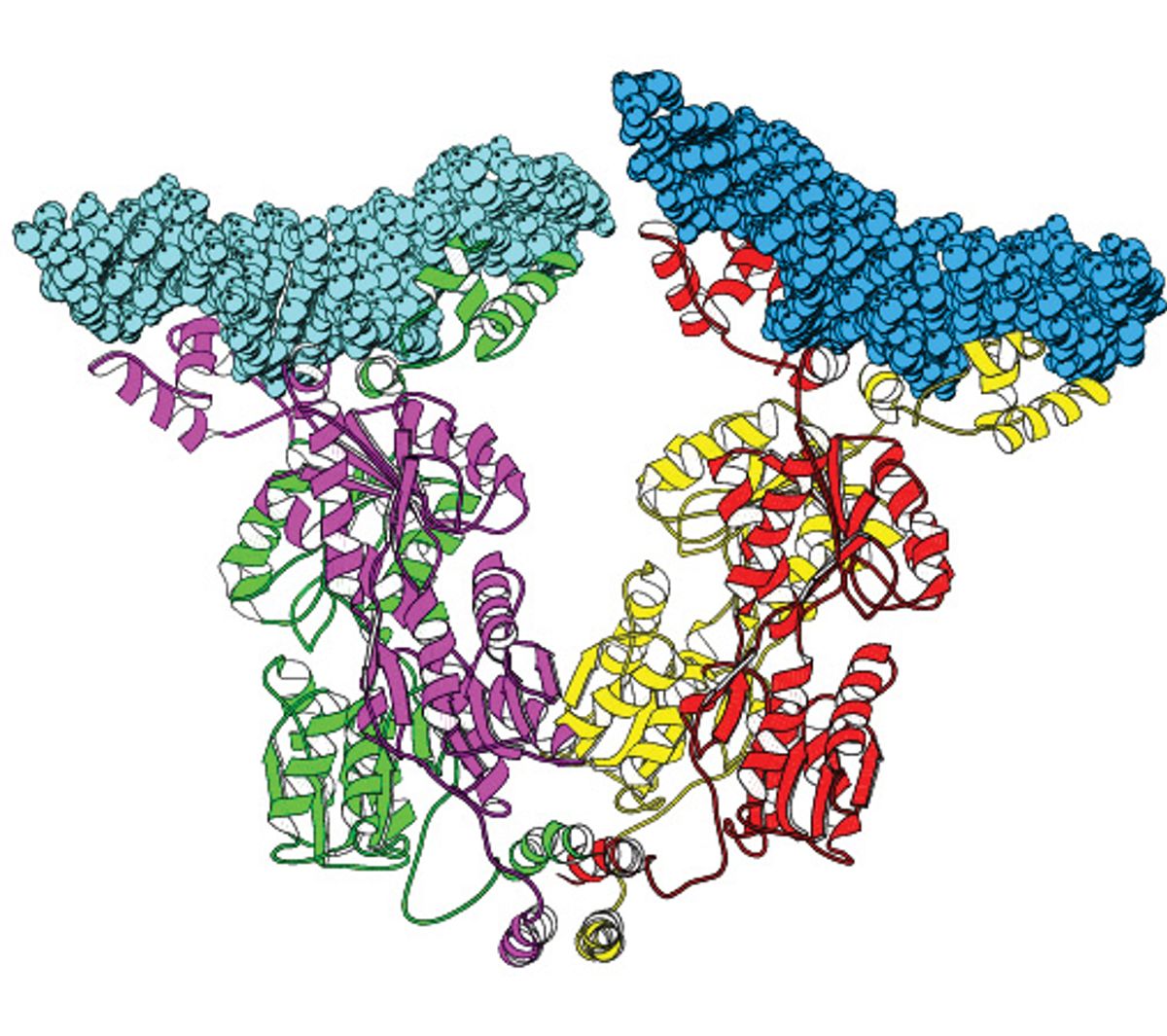
In 1977, Müller-Hill’s group found two additional operator sequences in the lac region.24 “There was this mystery: Why in the world are there extra binding sites for [LacI]?” said Jim Maher, a systems biologist at the Mayo Clinic College of Medicine and Science. He explained that most textbooks illustrate the lac operon as a linear construct of genes where LacI binds to the DNA to prevent gene expression by physically blocking the access of RNA polymerase. “That story is an oversimplification.”
Almost a decade after the discovery of the additional operator sequences, scientists proposed that the function of these extra regions was to increase lac repression by DNA looping.25,26 The repressor could bind directly to the primary operator by colliding with it, or, using the second set of DNA-binding subunits, LacI could bind to a distant site first and be brought to the main operator through this DNA architecture and bind this sequence as well. “You make a much tighter switch if there's multiple sources of this protein that can join there, and you don't need as much protein floating around to get the switch to go off and on,” Maher explained.
The Regulation of the lac Operon![]() modified from © istock.com, VanReeel, selvanegra, vdvornyk; stock.adobe.com, molekuul.be; designed by erin lemieux The genes and important sequences for the regulation of the lac operon are organized such that several mediators can fine tune expression of the three genes in the operon itself—lacZ, lacY, and lacA. The gene lacI, which encodes the lac repressor, LacI, sits outside the operon and is constitutively expressed. A cyclic adenosine monophosphate (cAMP) receptor protein (CRP) binding site (C) sits in front of the promoter region where there are two potential RNA polymerase binding sites (P1 and P2). CRP controls the binding of RNA polymerase between P1 and P2 by binding C. Finally, three operator regions, O1, O2, and O3, are spaced through the DNA region to modulate LacI binding and overall operon repression. Leaky Repression![]() modified from © istock.com, VanReeel, selvanegra, vdvornyk; stock.adobe.com, molekuul.be; designed by erin lemieux When glucose, but not lactose, is available in the cell, the LacI binds to the O1 sequence, preventing RNA polymerase from binding to the promoter region. However, due to protein kinetics, a small amount of the lac operon genes can be expressed if the polymerase binds when one repressor releases the DNA before another binds. Strong Activation![]() modified from © istock.com, VanReeel, selvanegra, vdvornyk; stock.adobe.com, molekuul.be; designed by erin lemieux If glucose becomes unavailable but lactose is present, then allolactose (grey sphere) binds LacI, releasing it from the operator. cAMP, produced in the absence of glucose, attaches to CRP, prompting its binding to the C site. This directs the RNA polymerase to sit on the P1 site, which promotes robust expression of the lac operon to facilitate lactose digestion. Tight Repression![]() modified from © istock.com, VanReeel; stock.adobe.com, molekuul.be; designed by erin lemieux The repression of the lac operon in the absence of lactose can be improved through DNA looping, in which LacI binds to O1 and a second operator sequence, either O2 or O3. This increases the local concentration of LacI, reducing transient expression that occurs when only free-binding lac repressor is available. Weak Activation![]() modified from © istock.com, VanReeel, selvanegra, vdvornyk; stock.adobe.com, molekuul.be; designed by erin lemieux When glucose and lactose are both available, allolactose releases LacI from the operator, allowing binding of the RNA polymerase. However, in the absence of cAMP to permit CRP binding, the polymerase binds either P1 or P2 and does not remain on the DNA as effectively, leading to low expression of the lac operon genes. |
Resolving the lac Repressor Structure: Regulation is Loopy
In the early 1990s, 30 years after Jacob and Monod’s discovery, the 3D structure of the lac repressor remained elusive. Mitchell Lewis, a structural biologist at the University of Pennsylvania, was a postdoctoral fellow at Harvard University under Mark Ptashne, a molecular biologist who identified the sequence and structure of the lambda bacteriophage repressor.27,28
At a celebration for this feat, Lewis told Gilbert that he intended to solve the structure of LacI. “He looked at me and said, ‘Mitch, bigger men than you have tried,’” Lewis recalled.
At the time, producing and purifying sufficient protein for X-ray crystallography was difficult, but advances in high-performance liquid chromatography improved the yield. Lewis and his team solved the structure of the tetramer alone, bound to the operator sequence, and bound to an allolactose analog.29 “That was the most exciting thing,” Lewis said. “You could actually see how this molecule switched from different conformations to either bind DNA or release the DNA.”
Subsequently, scientists solved crystal structures that shed light on the intricacies of DNA looping in lac repression.30,31 However, these intense investigations into the inner workings of a bacterial gene network do not exist in isolation. “The biggest thing was that, after [the discovery of a gene regulatory protein], people discovered that these transcription factors regulated gazillions of things,” Busby said.
“It's more than just bacterial genetics,” Lewis said about the importance of the lac operon. “It works in a slightly different way in eukaryotic systems, but the basic idea is you need to be able to turn genes on and off.”
For example, the expression of the gene sex-determining region Y (Sry) in many mammals activates a program involving several other genes to promote the development of male characteristics in the species.32 Additionally, understanding the transcriptional program in development identified the so-called Yamanaka factors that gave rise to induced pluripotent stem cells.33
Turning the Inducible lac Operon into a Tool
Once researchers established most of the inner workings of the lac operon, they began to use this system to their advantage. “Molecular biologists love tools,” Maher said. “When we need to turn genes off and on, which we often do in molecular biology, one of the first ways we do it is to turn to the lac operon.”
Using a lactose analog or replacing the lactose control with tetracycline, scientists use the principles of the lac operon to selectively control expression of genes of interest.34 Researchers even applied bacterial genetics directly to mammalian genetics, using the lac operon as a switch to control expression in a gene transfer experiment in mice.35
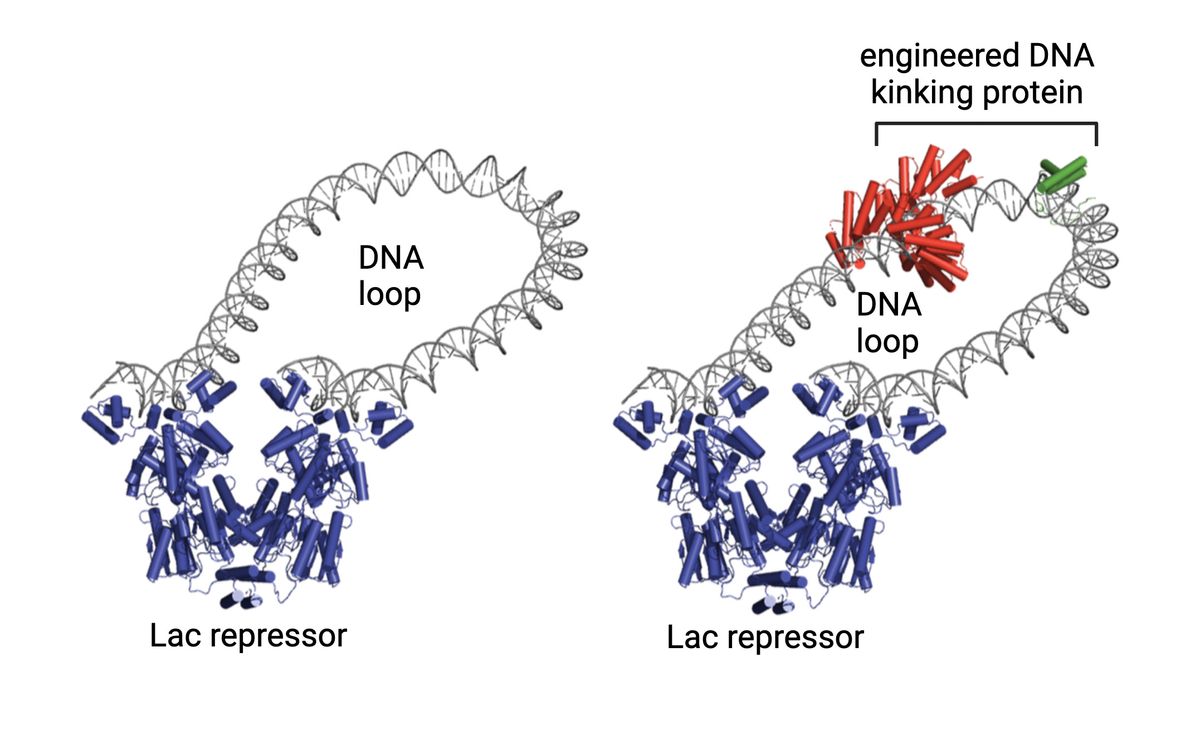
Many researchers are also applying knowledge from the lac operon to tangential systems and questions. For example, Maher uses the DNA architecture of the lac operon to understand how DNA makes switches and wraps around accessory proteins to regulate gene expression or for normal packaging. “We exploit that system, not because we're so interested in studying lactose biology, but we're very interested in the stiffness of this piece of intervening DNA,” he said. They are also interested in applying micro loops like those in the lac operon to eukaryotic systems to repress genes.
Today, the lac operon remains a mainstay in biology lectures as the paradigm for gene regulation. The regulatory system and its derivatives are often used as a jumping off point for industrial and therapeutic protein production, and scientists continue to improve upon these models.36–38 “It provides a way to leap off and say, ‘how can we use this information to develop new, modern switches that can be used in eukaryotic systems and mammalian systems?’” Lewis said.
- Monod J, Audureau A. Mutation and enzymatic adaptation in Escherichia coli-mutabile. Ann Inst Pasteur. 1946;72(11/12):868-878.
- Monod J, et al. Sur la biosynthese de la β-galactosidase (lactase) chez Escherichia coli. La specificite de l’induction. Acta Biochim Biophys. 1951;7:585-599.
- Monod J, et al. La cinétique de la biosynthèse de la β-galactosidase chez E. coli considérée comme fonction de la croissance. Acta Biochim Biophys. 1959;9:648-660.
- Jacob F. Transduction of lysogeny in Escherichia coli. Virology. 1955;1(2):207-220.
- Kaiser AD, Jacob F. Recombination between related temperate bacteriophages and the genetic control of immunity and prophage localization. Virology. 1957;4(3):509-521.
- Pardee A, et al. The genetic control and cytoplasmic expression of “Inducibility” in the synthesis of β-galactosidase by E. coli. J Mol Biol. 1959;1(2):165-178.
- Jacob F, Monod J. Genes determining the structure and regulatory genes in the biosynthesis of proteins. C R Acad Sci. 1959;249:1282-1284.
- Jacob F, et al. The operon: a group of genes with expression coordinated by an operator. C R Acad Sci. 1960;250:1727-1729.
- Jacob F, et al. The promoter, a genetic element necessary for the expression of an operon. C R Acad Sci. 1964;258:3125-3128.
- Miller JH, et al. The promoter-operator region of the Lac operon of Escherichia coli. 1968;38(3):413-420.
- Burstein C, et al. Role of lactose and its metabolic products in the induction of the lactose operon in Escherichia coli. Biochim Biophys Acta. 1965;95(4):634-639.
- Nakada D, Magasanik B. The roles of inducer and catabolite repressor in the synthesis of β-galactosidase by Escherichia coli. J Mol Biol. 1964;8(1):105-127.
- Jacob F, Monod J. Genetic regulatory mechanism in the synthesis of proteins. J Mol Biol. 1961;3(3):318-356.
- Jobe A, Bourgeois S. lac repressor-operator interaction: VI. The natural inducer of the lac operon. J Mol Biol. 1972;69(3):397-404.
- Pastan I, Perlman R. Cyclic adenosine monophosphate in bacteria: In many bacteria the synthesis of inducible enzyme requires this cyclic nucleotide. Science. 1970;169(3943):339-344.
- Maquat LE, Reznikoff WS. In vitro analysis of the Escherichia coli RNA polymerase interaction with wild-type and mutant lactose promoters. J Mol Biol. 1978;125(4):467-490.
- Beckwith J, et al. Evidence for two sites in the lac promoter region. J Mol Biol. 1972;69(1):155-160.
- Malan TP, McClure WR. Dual promoter control of the escherichia coli lactose operon. Cell. 1984;39(1):173-180.
- Straney DC, et al. Synergy between Escherichia coli CAP protein and RNA polymerase in the lac promoter open complex. J Mol Biol. 1989;206(1):41-57.
- Malan TP, et al. Mechanism of CRP-cAMP activation of lac operon transcription initiation activation of the P1 promoter. J Mol Biol. 1984;180(4):881-909.
- Gilbert W, Müller-Hill B. Isolation of the lac repressor. Proc Natl Acad Sci USA. 1966;56(6):1891-1898.
- Müller-Hill B, et al. Specificity of the induction of the enzymes of the lac operon in Escherichia coli. J Mol Biol. 1964;10(2):303-318.
- Gilbert W, Maxam A. The nucleotide sequence of the lac operator. Proc Natl Acad Sci USA. 1973;70(12):3518-3584.
- Kania J, Müller-Hill B. Construction, isolation and implications of repressor-galactosidase ·ß-galactosidase hybrid molecules. Eur J Biochem. 1977;79(2):381-386.
- Oehler S, et al. The three operators of the lac operon cooperate in repression. EMBO. 1990;9:973-979.
- Mossing MC, Record MT. Upstream operators enhance repression of the lac promoter. Science. 1986;233(4766):889-892.
- Hochschild A, et al. Repressor structure and the mechanism of positive control. Cell. 1983;32(2):319-325.
- Anderson JE, et al. Structure of the represser–operator complex of bacteriophage 434. Nature. 1987;326(6116):846-852.
- Pace HC, et al. lac repressor: Crystallization of intact tetramer and its complexes with inducer and operator DNA. Proc Natl Acad Sci USA. 1990;87(5):1870-1873.
- Lewis M, et al. Crystal structure of the lactose operon repressor and its complexes with DNA and inducer. Science. 1996;271(5353):1247-1254.
- Friedman AM, et al. Crystal structure of the lac repressor core tetramer and its implications for DNA looping. Science. 1995;268(5218):1721-1727.
- Larney C, et al. Switching on sex: Transcriptional regulation of the testis-determining gene Sry. Development. 2014;141(11):2195-2205.
- Takahashi K, Yamanaka S. Induction of pluripotent stem cells from mouse embryonic and adult fibroblast cultures by defined factors. Cell. 2006;126(4):663-676.
- Skerra A. Use of the tetracycline promoter for the tightly regulated production of a murine antibody fragment in Escherichia coli. Gene. 1994;151(1-2):131-135.
- Hu MC-T, Davidson N. The inducible Iac operator-repressor system is functional in mammalian cells. Cell. 1987;48(4):555-566.
- Maccormick CA, et al. Construction of a food-grade host/vector system for Lactococcus lactis based on the lactose operon. FEMS Microbiol Lett. 1995;127(1-2):105-109.
- Graumann K, Premstaller A. Manufacturing of recombinant therapeutic proteins in microbial systems. Biotechnol J. 2006;1(2):164-186.
- Lalwani MA, et al. Optogenetic control of the lac operon for bacterial chemical and protein production. Nat Chem Biol. 2021;17(1):71-79.